When it comes to microscopy, scientists want to get images fast – in just a matter of seconds. But despite all of the advantages of broadband CARS, the technique is still a bit on the slow side.
Unlike many approaches to imaging biological specimens and other materials, such as fluorescence microscopy, coherent anti-Stokes Raman scattering (CARS) does not require any sample staining or chemical tagging, both of which can be invasive.
The method is based on two laser pulses (pump and Stokes), in which the difference between the two wavelengths is matched in resonance with specific molecular vibrational modes. The light interacts with the sample and generates a shorter-wavelength (anti-Stokes) pulse that provides information about the sample’s chemical composition. By scanning the laser beams, a chemically specific 3-D image can be obtained that describes the concentration of the excited molecular oscillators within the sample.

A CARS hologram can be recorded in a single shot, opening up the possibility of 3-D imaging of fast dynamical phenomena at laser-pulse-width-limited speed. Courtesy of Chuan Yang, Perry Edwards and Dr. Zhiwen Liu, Penn State University.
CARS has found a niche in the bio-imaging community because of its inherent chemically selective capability – it is label-free – and its typically orders-of-magnitude enhancement of the CARS signal in comparison with standard spontaneous Raman. Specifically, it has been shown to be a viable method for imaging lipids and detecting bacteria spores. It also has the potential to be realized in various types of biochemical sensors and used for the detection of trace chemicals in remote-sensing platforms.
Narrowband, or single-frequency, coherent Raman spectroscopy is a well-established method that is faster than broadband CARS, but it cannot obtain fingerprint spectra required for highly selective chemical imaging. For this, broadband CARS is required, but progress here is slower.
The reason is that there is an upper limit to the amount of signal one can extract, said Dr. Marcus T. Cicerone, project leader in the Polymers Division at the National Institute of Standards and Technology in Gaithersburg, Md. And in broadband CARS, the signal is spread over a very wide bandwidth (~3000 cm—1, as compared with ~10 cm—1 for narrowband coherent Raman). On the other hand, it is much more powerful, offering significantly more chemical selectivity.
“Work in spontaneous Raman spectroscopy has shown that broadband Raman (particularly fingerprint Raman spectra) can be used to diagnose some diseases with more than 95 percent sensitivity and selectivity,” Cicerone said. “Because of the exquisite chemical sensitivity of broadband Raman scattering, the list of what it can detect is almost endless.”
Why, then, is spontaneous Raman hardly ever used? The answer is that it is too slow and inefficient. Furthermore, there is little hope of fundamental improvements in speed with spontaneous Raman, Cicerone believes. Broadband coherent Raman methods, on the other hand, do present opportunities for improvement in signal acquisition.
Work by Cicerone and others, such as Hiro-o Hamaguchi at the University of Tokyo, has already shown a fiftyfold increase in spectral acquisition rates for broadband CARS, but there is room for even more significant improvements.
“I think that speeding up broadband coherent Raman signal acquisition is the most important and exciting potential event in the field,” Cicerone said. “Speeding broadband signal acquisition will allow people to take advantage of the decades of spontaneous Raman spectroscopy work to get exquisite chemical selectivity and sensitivity in a spatially resolved way – and very rapidly, with no staining or special sample preparation.”
Holography could be an answer
By capturing a hologram of the complex CARS image field, the combination of CARS and holography could be one way of speeding up image acquisition. An advantage of CARS holography is that it can alleviate the need for laser scanning: The hologram can be used to digitally reconstruct and back-propagate the complex CARS field and therefore digitally focus onto different depths inside the sample, providing 3-D chemically specific information.
A team of researchers, Dr. Zhiwen Liu, Dr. Kebin Shi, Perry Edwards and colleagues at Penn State University in University Park, Pa., and Dr. Demetri Psaltis at École Polytechnique Fédérale de Lausanne in Switzerland, notes that existing CARS microscopy techniques suffer from an inherent limitation of sample or laser scanning.
Similarly, in wide-field CARS imaging, axial scanning is still required to focus to different depth positions inside a sample because only the intensity of the CARS signal is recorded each time.
“The limitation on speed comes from the methods used to acquire CARS images, such as the scanning approach to form an image of the sample of interest,” Edwards said.
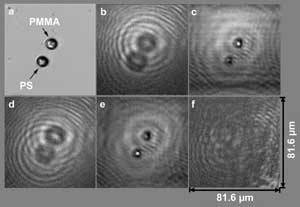
Chemical-selective in-line holographic CARS imaging. (a) An optical microscope image of PMMA (polymethyl methacrylate) and a polystyrene (PS) sphere; (b) a hologram recorded at PMMA resonance; (c) reconstruction by digital back-propagation showing a resonant PMMA microsphere; (d) hologram recorded at polystyrene resonance; (e) reconstruction by digital back propagation showing a resonant polystyrene microsphere; (f) a hologram recorded without the use of a nonlinear layer. Figure reprinted with permission from Qian Xu et al (2010). Inline holographic coherent anti-Stokes Raman microscopy. Opt Exp, Vol. 18, pp. 8213-8219.
CARS is ideally suited for recording a hologram because it is a four-wave mixing process that produces a coherent signal, Liu explained.
“CARS holography thus combines the chemical-selective capability of CARS with the nonscanning 3-D imaging capability of holography to establish a new technique for chemical and biological imaging,” Edwards said. “We believe that CARS holography can be a powerful tool for studying dynamic biological, physical and chemical processes due to its high speed and sensitivity.”
In the setup, molecules of interest within a sample specimen are excited with an incident near-infrared pump/probe laser and a Stokes laser beam. This generates an anti-Stokes signal, which is of a shorter wavelength and is blue-shifted.
The Stokes beam is tuned to resonantly excite the particular molecules of interest via the CARS process, and the resulting anti-Stokes signal is detected by an imaging device.

Chemical-selective CARS holographic imaging. (a) Single-shot CARS hologram recorded at polystyrene resonance wavelength; (b) single-shot hologram recorded at PMMA resonance wavelength; (c) reconstructed CARS intensity image from the hologram shown in (a); (d) reconstructed CARS intensity image from the hologram shown in (b). Figure reprinted with permission from Kebin Shi et al (March 2010). Coherent anti-Stokes Raman holography for chemically selective single-shot nonscanning 3D imaging. Phys Rev Lett, pp. 093902-093905. http://prl.aps.org/abstract/PRL/v104/i9/e093902). ©2011 by the American Physical Society.
Also incident on the imaging device is a plane-wave reference beam
matched to the same frequency as the generated CARS signal. The CARS
signal and the reference beam interfere to generate a fringelike pattern
that is recorded on the imaging device. It is this recorded image that
represents a hologram and contains both the amplitude and the phase
information of the anti-Stokes signal.
Finally, a Fourier transform is performed on the hologram, and the relevant signal is filtered and extracted by taking the inverse Fourier transform. This extracted signal, containing both amplitude and phase components, represents the nondiffraction-compensated image. By using digital propagation via a diffraction algorithm, CARS images at different propagated planes can be digitally realized.
“In our work, CARS holograms with an exposure time limited by laser-pulse duration time (~5 ns) were demonstrated,” Liu said. “Since a CARS hologram can be recorded in a single shot, it opens the possibility of three-dimensional imaging of fast dynamical phenomena at laser-pulse-width-limited speed.”
Additionally, the holographic CARS technique was boosted by recent work on compressive holography carried out by professor David Brady’s group at Duke University in Durham, N.C., which showed that tomographic reconstruction also can be obtained from a hologram, if the objects are sparsely distributed.
What’s more, the presence of an intense reference beam (i.e., a local oscillator) and the coherent homodyne detection nature of CARS holography also could lead to shot-noise-limited detection sensitivity.
Superresolution
Besides increasing the speed (temporal resolution) of CARS, it is important to improve its spatial resolution. Although superresolution now can be accomplished in far-field fluorescence microscopy through techniques such as STED (stimulated emission depletion microscopy) and STORM (stochastic optical reconstruction microscopy), an interesting challenge facing scientists is whether methods can be developed to achieve superresolution in far-field CARS or stimulated Raman scattering imaging.
CARS and stimulated Raman scattering imaging systems require more complex laser systems than do other imaging modalities. This means that improvements made to optical technologies in laser systems – and a reduction in their cost and complexity – will be crucial to widening the accessibility of CARS and stimulated Raman scattering to biologists and other researchers.