Whole-animal imaging has become an indispensable tool, enabling researchers to noninvasively identify a disease and its location within an animal subject, to monitor disease development over time and to highlight underlying mechanisms through application of disease-associated biomarker-targeted agents.
Optical imaging of small animals has moved beyond simply determining whether a tumor is growing or regressing in response to a therapy. Today, it can be used to track the biodistribution of labeled cells and compounds, and for preclinical toxicity testing; and to better understand the mechanism by which a tumor or disease progresses. The ability to image deeper with improved clarity, resolution and sensitivity is redefining the limits of whole-animal imaging.
(1) In vivo image of two hydra using quinaldine red (QR) fluorescence labels from the tentacles (t) to the peduncle (p) located in the upper part of the image. An adult (a) with a bud (b) on the left side turns the hypostome (h), surrounded by a ring of tentacles, toward the camera. (2) In vivo image of different polyps. In the foreground, a hydra turns the hypostome toward the bottom. A strong punctuated fluorescence labels the mouth, the tentacles and, at a lower extent, the animal body. Scale bar = 500 µm. Courtesy of Claudia Tortiglione.
Imaging whole animals is essential to better understand cancer, diabetes, and infectious, cardiovascular, immunological and neurodegenerative diseases, among others.
Although the major applications remain the tracking of diseases, highlighting their pathways and testing various therapeutic interventions, other research areas are increasingly adopting imaging as a critical research tool. These include stem
cells and regenerative medicine, cardiovascular disease and neurology. And another emerging trend uses in vivo molecular imaging techniques as an early indicator of drug safety across all therapeutic areas.
“Such approaches have enhanced therapeutic development outcomes, evidenced by the contribution of imaging technologies to several cancer and infectious disease treatments in the market and in clinical trials,” said Dr. Vivek Shinde Patil, senior manager of technical applications at human and environmental health specialist Perkin-Elmer Inc. of Waltham, Mass. “Indeed, imaging has changed the paradigm of preclinical research by reducing animal usage in biomedical research, improving study outcomes through superior statistics and tighter controls, and increasing speed and efficacy of therapeutic evaluations.”
Tracking N. vectensis development by QR labeling up to 21 days postfertilized. Courtesy of Ambrosone et al, Nanotoxicology 2013; p.f. = postfertilization.
Trend 1: 3-D imaging
Optical imaging relies on the detection of light-producing reporters – as in bioluminescence, chemiluminescence and fluorescence – to provide functional and molecular readouts. Advances in these techniques as well as an increased trend to combine methodologies are allowing researchers to explore multiple physiological outcomes within the same animal, in parallel.
A key trend driving the in vivo imaging industry is a move away from 2-D imaging, which can be used to identify the location of a major organ as an anatomical frame of reference, to 3-D reconstructive multimodal imaging.
This involves co-registering, or combining, optical imaging with other techniques such as microcomputed tomographic (microCT) imaging, MRI, positron emission tomography (PET) or single-photon emission computed tomography (SPECT).
PerkinElmer’s IVIS SpectrumCT instrument combines fluorescence, bioluminescence, radioisotopic Cerenkov and microCT imaging; it is part of the IVIS product line that includes the Kinetic, Lumina II, XR and Spectrum. The line has been used in stem cell studies for tissue engineering, cancer studies and other imaging research.
Trend 2: Fluorescence vs. bioluminescence
Over the past five years, there has been a gradual shift in interest from bioluminescence imaging toward fluorescence imaging. Fluorescence imaging allows researchers to develop clinical applications of in vivo imaging such as disease detection, and progression and monitoring of drug efficacy.
At Li-Cor Biosciences of Lincoln, Neb., which designs and manufactures instrument systems for environmental and biotechnology research, the Pearl Impulse product line has introduced FieldBrite technology, which the company says is a game changer for fluorescence optical imaging.
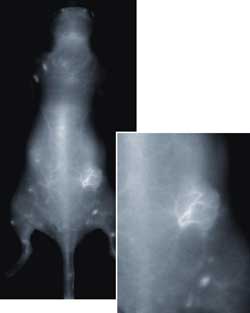
Surface vasculature imaged using Pearl Impulse after IV injection. Tumor was implanted on the right flank of nude mouse. Increased vasculature is seen in the tumor region (inset). Courtesy of Li-Cor.
“With six log dynamic range of our system, it is impossible to obtain saturation of signal,” said product manager Jeff Harford. “This means that the same camera settings can be used for all studies, simplifying the longitudinal comparison of images. It also makes it very simple to work with, as all you need to do is snap a picture with the click of a single button.”
This is not possible using traditional cameras for which you would have to capture images of the same animal over a longitudinal study using several different camera settings. This makes it tricky to assess what is really going on in the animal.
Trend 3: Red and near-IR wavelengths
This is really a trend within a trend: Including the increasing adoption of fluorescence imaging, there is also a move toward the red and near-IR wavelengths for deeper imaging penetration.
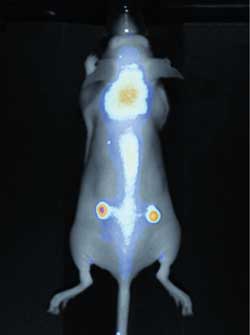
Subcutaneous tumors were detected in a nude mouse using Li-Cor’s Pearl Impulse. Courtesy of Li-Cor.
The introduction of new genetically encoded and dye-labeling technologies in the 700- to 900-nm range has helped boost the reach and popularity of fluorescence imaging; e.g., the Li-Cor IRDye 800CW dye is designed for the IR.
A key advantage of dyes in this region of the spectrum is that light absorption and scattering by endogenous chromophores present in living tissues – such as hemoglobin, melanin and lipids – decrease above 700 nm; tissue autofluorescence is minimal at 800 nm. This means that background noise will be minimized in this spectral region, yielding high signal-to-noise ratios.
“The ability to image deeper within the animal with improved clarity, resolution and sensitivity [is] also redefining the limits of whole-animal imaging,” Patil said. “Such advances have enabled exploration of genetic-level ‘molecular’ activity in whole animals and visualization of anatomic structures down to unprecedented levels noninvasively.
“These techniques are also becoming faster and multimodal, thereby facilitating the complementary insights and higher correlative power stemming out of the combined use of multiple imaging modalities.”
Trend 4: Novel probes
Nanotechnologies provide a wide class of new probes garnering interest from scientists for their potential as highly effective diagnostic and therapeutic tools in one.
Claudia Tortiglione, one of the scientists investigating the capabilities of novel imaging probes, heads up the NanoBio-molecular group at the Institute of Cybernetics of CNR (National Research Council) in Pozzuoli, Italy.
“Based on their chemical composition, they display unique optical and physical properties spanning from fluorescence (semiconductor materials – i.e., quantum dots), to magnetism (iron oxide nanoparticles),” she said. “The possibility to decorate their surface with biomolecules (peptides, antibodies) makes these new probes capable to target a specific cell population, while the addition of nucleic acids or drugs add the capability to deliver bioactive molecules, transforming the imaging probes from diagnostic to therapeutic tools.”
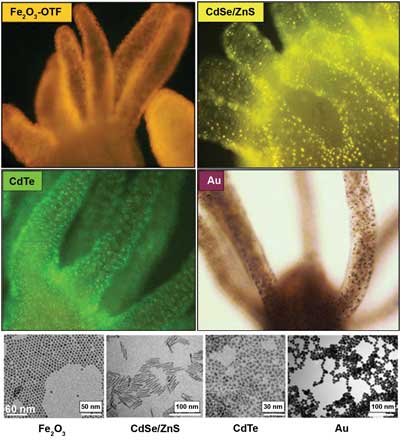
Labeling hydra with nanocrystals. Upper left panel: a living hydra incubated with bifunctional conjugates (Fe2O3-OTF [oligothiophene fluorophores]). Upper right panel: a hydra labeled with fluorescent rod-shaped nanocrystals. Lower left panel: CdTe QD aminos stabilized by cysteamine were added to the medium bathing living hydras and imaged after four hours. Lower right panel: a hydra treated with polyethylene-glycol-coated gold nanoparticles. Bottom: representative transmission electron microscopy images of the samples above. Courtesy of Claudia Tortiglione.
In “Imaging inward and outward trafficking of gold nanoparticles in whole animals,” a March 2013 American Chemical Society Nano paper, Tortiglione and colleagues investigated the dynamic of internalization of gold nanoparticles at an ultrastructural level (transmission electron microscopy).
The group discovered previously unknown biological routes when it tracked gold nanoparticles on their way in and out of the cells in a simple water invertebrate. This provides dynamic and kinetic clues that the team said are important to take into account when designing nanomaterials to interfere with biological samples.
The researchers also confirmed the use of gold nanoparticles as a novel, safe and biocompatible tool for biological imaging, clinical diagnostics and therapeutics.
Challenges
Imaging at the whole-animal level allows us to investigate in situ how tissue cells undergo cell renewal, migration and differentiation, in their physiological context and without the effects of external manipulation.
But light transmission through intact biological tissue is complex because it suffers from scattering-dominated attenuation, heterogeneous physical properties as well as the presence of endogenous absorbers and chromophores in animal tissues.
“Three-dimensional reconstruction of optical sources within whole animals in order to accurately quantify their location and activity therefore needs to take these effects into account,” Patil said. “Significant improvements in hardware design, light-propagation models and image processing algorithms have allowed us to make great strides in 3-D optical imaging.”
New imaging probes also pose a risk in terms of potential toxicity in cells. Besides the in vitro trials, the toxicity may occur in vivo, and it may depend on the administration route (topical, systemic delivery or injection) as well as on the biodistribution, retention and excretion time following body entrance.
Tortiglione highlights the need for toxicological evaluation of these new probes to be extended to in vivo trials. “It is known that physical parameters such as size, charge, chemical composition trigger specific cell responses,” she said. “However, toxicological studies have been performed by different groups using different cell lines, dose and concentrations – different chemical protocols even – to test the same materials, and, to date, a uniform evaluation of the potential toxicity of a given nanoparticle for human purposes is missing.”