A digital micromirror device serving as the spatial light modulator can overcome shortcomings of traditional spectroscopy architectures.
MIKE WALKER, TEXAS INSTRUMENTS INC., AND HAKKI REFAI, OPTECKS LLC
In the field of near-infrared (NIR) spectroscopy, a system that combines portability with the accuracy and functionality of high-performance laboratory systems would significantly enhance real-time analysis. The development of small handheld spectrometers, powered by a battery, could lead to more efficient monitoring of industrial processes or assessments of food ripeness in the field.
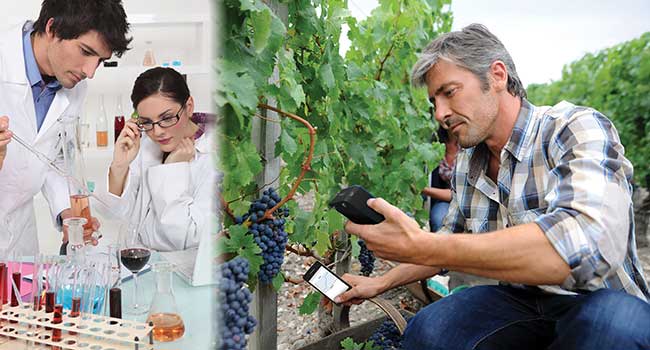
Spectroscopy is used to evaluate the chemical makeup of items in the lab and the field. Courtesy of Texas Instruments.
Most dispersive IR spectroscopic measurements begin in the same manner. The light to be analyzed passes through a small slit that, combined with a grating, controls the resolution of the instrument. The diffraction grating is designed specifically to reflect light of different wavelengths at known angles. This spatial separation of wavelengths allows other systems to measure the light intensity on a wavelength-by-wavelength basis.
Traditional architectures for spectroscopic measurements differ mainly in how the dispersed light is measured. Two very common methods are 1) a single element (or point) detector combined with physical scanning of the dispersed light, and 2) imaging the dispersed light onto an array of detectors.
Approach using MEMS technology
Many limitations of traditional spectroscopy methods can be overcome using a new approach based on optical microelectromechanical systems (MEMS) array technology with a single-point detector. A solid-state optical MEMS array replaces the traditional motorized grating in a single-point detector-based system with a simple, spatial wavelength filter. This approach can utilize the performance advantages of a single-point detector while eliminating the issues of a finely controlled motorized system. In recent years, such systems have been produced in which the scanning grating is eliminated and the MEMS device filters each specific wavelength into a single point detector. This method has demonstrated high performance while resulting in a more compact and robust spectrometer.

A spectrometer is used to analyze pharmaceuticals. Courtesy of Texas Instruments.
The use of an optical MEMS array has several advantages over a linear array detector architecture. First, a larger, single element detector can be used to increase light collection and greatly reduce system cost and complexity, especially for infrared systems. Also, by eliminating the array detector, the pixel-to-pixel noise is eliminated, which can be a significant improvement in the signal-to-noise ratio (SNR) performance. The increase in SNR allows more accurate measurements to be obtained in less time.
In a spectroscopy system using MEMS technology, the diffraction grating and focusing elements perform the same functions as before, but now light from the focusing element is imaged onto the MEMS array. To select a wavelength for analysis, a specific band of spectral response is activated so as to direct light to the single point detector element for collection and measurement.
The advantages are realized if the MEMS device is highly reliable and can produce a filter response that is predictable and constant over time and temperature.
Using a digital light proccessing (DLP) chip or digital micromirror device (DMD) as a spatial light modulator can overcome several challenges if used as the MEMS device in a spectrometer system architecture. First, light is switched on and off into the single point detector using an array of mirrors composed of aluminum, which is optically efficient over a wide range of wavelengths. Second, the on and off states of digital mirrors are controlled by mechanical stops and a latched circuit of complementary metal oxide semiconductor (CMOS) static random access memory (SRAM) cells, providing fixed voltage mirror control. This fixed voltage static control means the system does not require a mechanical scanning or analog control loop and can simplify calibration. It also makes the spectrometer design more resistant to sources of error such as temperature, aging or vibration.
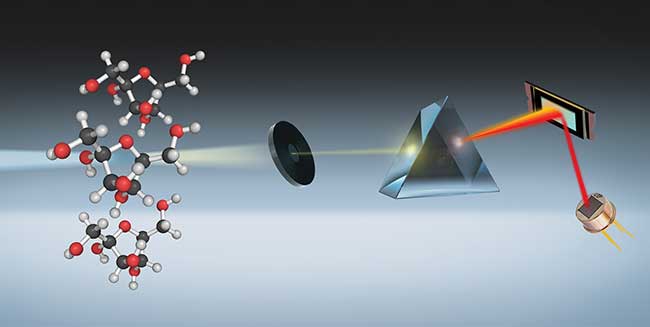
An illustration of how spectroscopy works with DLP technology. Courtesy of Texas Instruments.
The programmable nature of the DMD has many advantages. One advantage occurs when designing a spectrometer architecture based on addressing columns of mirrors as a filter. Since the resolution of the DMD is typically higher than the desired spectrum, the DMD area can be underfilled and the spectrum can be oversampled. This enables wavelength selection to be fully programmable and allows for extra micromirrors to be used as recalibrated columns in case of extreme mechanical shifts of the optical engine.
Also, the DMD is a two-dimensional programmable array, which provides the user a high degree of flexibility. Resolution and throughput can be adjusted by selecting a different number of columns. Scan time can be varied dynamically so that wavelengths of interest can be examined longer and in greater detail than wavelengths of little interest, making better use of instrument time and capabilities. Additionally, advanced aperture encoding techniques, such as applying Hadamard patterns, allow a high degree of flexibility and increased performance as compared to fixed filter implementations1.
In summary, spectroscopic devices using a DMD can enable higher resolution, higher flexibility, higher robustness, a smaller form factor and lower cost than current spectroscopy systems, making them attractive for a broader range of commercial and industrial applications.
Single detector architecture eliminates noise
Today’s linear array-based spectrometer is limited by two primary factors. First, the wavelength selection presented to the detector is constrained by the pixel aperture. The size of a detector determines the amount of light collected and bounds the SNR. A typical indium gallium arsenide (InGaAs) 256-pixel linear array, such as Hamamatsu G9203-256, is 50 × 500 µm. Conversely, an array of digital micromirrors is a fully programmable matrix where the number of columns and scan technique can be configured for applications. This allows a larger signal to be presented into a larger 1- or 2-mm single point detector typically used with a DMD. Filtering narrow bands of light into a linear array, which are typically 50-µm-wide pixels, may present challenges of crosstalk. Pixel-to-pixel disturbances can become the primary contributor to noise in the reading. These disturbances are eliminated by the single detector architecture. Also, by taking advantage of the digital micromirror scan speeds of 1 kHz to 4 kHz, single point scans can reach similar dwell times to parallel multipoint sampling. Results have shown SNR ranges >10,000:1 for compact spectrometer engines based on MEMS (or based on a DMD).
2D MEMS array key to mobile spectrometer
In order to maximize performance, the user needs to consider the overall MEMS area that can be used to reflect light into the detector. This can then be carefully matched to available single point detector aperture sizes.
A DMD exists that utilizes a 5.4-µm micromirror with over 400,000 available pixels and is optimized for wavelengths of 700 to 2500 nm. That DMD is the DLP2010NIR, which utilizes a new pixel architecture know as TRP. This pixel, seen in Figure 1, provides a 17° tilt angle. DLP2010NIR was implemented in an evaluation module that provides a unique optical architecture for spectroscopy use cases. Using an optical path that leverages the 17° on and off angles enables a high-performance sensing solution with a compact engine that minimizes stray light.

Figure 1. A micromirror structure with a 17° tilt angle. Courtesy of Texas Instruments.
An illustration of this unique optical engine for spectroscopy use cases is seen in Figure 2. The system optimizes optical signals throughout the light path. Response from the sample is imaged onto the DMD, allowing spatial control of each wavelength. The evaluation module is intended to capture the design advantages of using a highly efficient MEMS as a high-speed 2D filter for spectroscopy. It is a compact, rugged and highly adaptable system capable of moving spectroscopic analysis out of the laboratory and directly to the field or source needing measurement. The ability to interchange measurement heads between transmissive and reflectance with the same device can enable performance benchmarking versus traditional spectrometers.
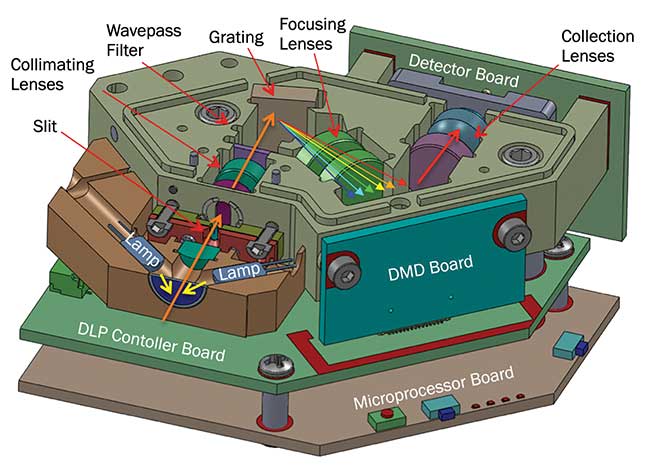
Figure 2. A spectrometer optical engine using a digital micromirror device (DMD) and single point detector. Courtesy of Texas Instruments.
There are several illumination modules for a spectroscopic optical engine that leverage the DLP2010NIR chip, and each works in slightly different ways. In a transmission module, a light source, cuvette holder, precision cuvette and additional mounting hardware are used to facilitate the measurement of absorption and scattering properties of a transmissive sample. NIR transmittance measurements can be used for liquid samples, such as water content or presence of gaseous signatures in juices. The data can provide a wealth of information about the juices’ origins. In solid samples, NIR transmittance can measure the opacity of plastic tubing, which is an important parameter in observing flow in gas and liquid delivery lines. In-line transmittance measurements are also used to analyze the water content of butter during production, which allows for timely adjustments to the butter-making process that saves time, minimizes costs and increases the quality of the end product.
Alternatively, a reflection module can be used for measurements that do not require the sample to be in contact with the spectrometer window. It gives flexibility to perform scans from several centimeters distance, such as monitoring meat quality after being wrapped in plastic. Health aspects such as blood glucose prediction can also be characterized in the NIR region using diffuse reflectance from skin.
Finally, in a fiber-coupled module, either transmittance or reflectance measurements are made via an optical fiber. This allows measurements when direct contact between the spectrometer and sample is not practical or possible. Examples of such sampling include monitoring industrial processes; measuring fluids as they are piped between vessels; and analyzing moisture fat and protein content in chicken, beef and pork. The modules greatly extend the range of applications and can provide enhanced measurement performance. Optecks has illumination module solutions that enable all of these sampling methods.
As discussed, spectroscopy devices using a DMD expand the ability to analyze, test and measure a multitude of substances. They provide a path to more accurate performance, higher resolution, more flexibility, improved robustness and smaller form factor light-sensing solutions. In addition, spectrometers using a DMD can bring increased measurement reliability that may not have been there before using traditional spectroscopy systems. Whether a user is looking to test how much water is needed for his crops on a farm, or he is trying to predict spoilage in food, spectroscopy continues to be a powerful approach for accurate, real-time analysis.
Reference
1. E. Pruett (April 2015). Latest developments in Texas Instruments DLP near-infrared spectrometers enable the next generation of embedded compact, portable systems. SPIE, Vol. 9482-13.
Meet the authors
Mike Walker is a business development manager for Texas Instruments’ DLP Products, leading its spectroscopy business. Prior to this role, Mike spent more than 30 years leading multiple technical and business teams at Texas Instruments; email: [email protected]. Hakki Refai, Ph.D., is chief technology officer for Optecks. Refai has more than 10 years of experience in the design and development of optical, electronic and software systems for DLP-based systems; email: [email protected].