Innovations in spectral-based real-time imaging lead to new applications in the operating room.
RICHARD MEESTER AND SANDER DE JONGE, QUEST MEDICAL IMAGING BV
In the last decade, researchers have worked extensively on medical devices and applications that measure the spectral response of tissue to various wavelengths of light. These applications, in a lab or proof-of-principle setup, have proven the usefulness of spectral imaging to strengthen diagnosis by uncovering information hidden in the spectral signature. Studies have proven that spectral signatures can distinguish between healthy and cancerous tissues. In particular, studies and EU-funded programs have been performed on brain cancer.
Usually the devices employed are slow, have low spatial resolution, high wavelength resolution and are physically bulky. Often these devices rely on touching the tissue to reduce false information or stray light coming from unintended sources. But this is not always possible because, depending on tissue type, damage may occur.
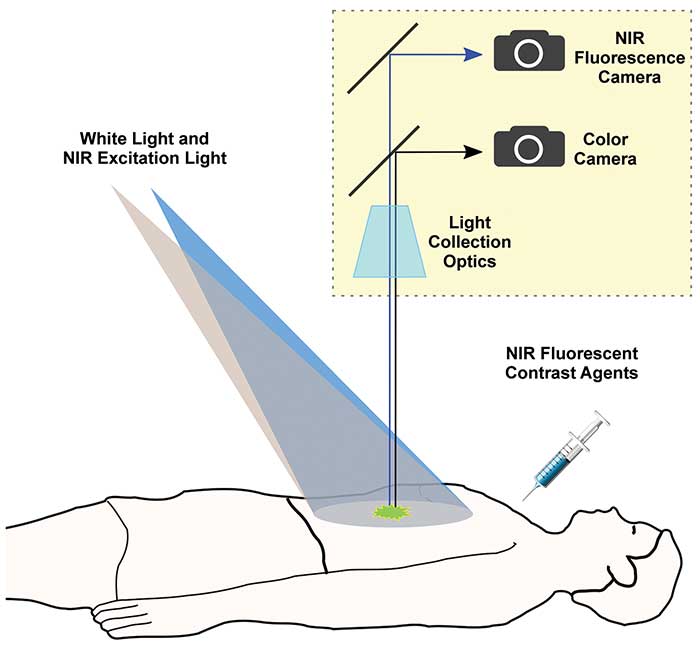
With fluorescence image-guided surgery, multiple cameras pick up images through a single lens. The cameras pick up different wavelengths of light originating from imaging agents inside tissue or the bloodstream, while at the same time acquiring 2D visible light images. Courtesy of Quest Medical Imaging.
The challenge has been to develop spectral imaging devices that work in a surgical setting to improve patient outcome. But the technology, which makes use of infrared imaging, is beginning to live up to its potential as an aid to surgeons. The use of spectral imaging devices to measure tissue perfusion and for lymph node mapping is becoming part of the standard toolset for surgeons. This adoption, in turn, is paving the way to more advanced applications of fluorescent agents in the surgical suite, from visualizing tumor margins to highlighting nerves and analyzing blood flow.
Fluorescence image-guided surgery employs multiple cameras or sensors through a single lens. The cameras or sensors pick up different wavelengths of light originating from imaging agents inside tissue or the blood stream, while at the same time acquiring 2D visible light images.
With this surgical procedure, an imaging agent is visualized by illuminating the tissue with LED or laser light of a wavelength and intensity that matches the contrast agent. This allows the agent to fluoresce and send out a Stokes shifted signal that is picked up by the camera system. Current applications are based on an imaging agent such as indocyanine green (ICG) that has no capabilities to “attach” or “grab” specific tissue and as a result is “free floating” in mediums such as arteries and veins, lymph tracks and micro vasculature.
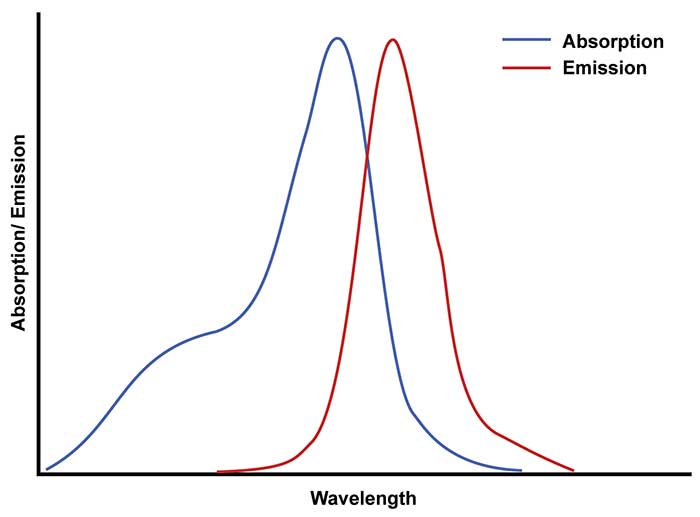
The chart shows the excitation wavelength of ICG (blue) and the emission wavelength (red) when laser light is applied. The difference between the two is what’s known as Stokes shift. Courtesy of Quest Medical Imaging.
The technology can be used during colon surgery in which anastomosis is performed. After a section of a colon is removed and the two ends are stitched back together, various complications can emerge that are difficult to assess by just the surgeon’s eyes. One is an anastomosis leak, which can cause peritonitis. A second is stitching that is too tight, which can result in dying tissue, colon perforations and other acute risks for the patient’s health because of insufficient blood perfusion.
Breast reconstruction or plastic surgery is another example where ICG is injected into the blood stream and perfusion can be assessed. As with the colon anastomosis, a good blood flow is imperative to the healing process of tissue. Any blockage, tight stitches or other problems with restoring a correct blood flow after the surgery can lead to complications.
To check for such problems using fluorescence, a contrast agent is injected into the blood stream after the tissue is surgically reconnected, immediately prior to visualization. The agent lights up when the excitation light is applied and captured by the camera. The surgical camera acquires two separate images; the first image is a colored visible light image, and the second is a grayscale infrared image of where the imaging agents light up.
When these images are placed on top of each other, the surgeon can see a high brightness where the dye is present and a dark signal where it is not. If the dye is seen leaking from the tissue, this indicates that the wound is not closed correctly. If stitches are too tight and the blood flow is blocked, the problem shows up as a dark region where a bright “glow” in the tissue is expected.
Employing fluorescence imaging for lymph node mapping during breast cancer surgery is currently an off-label use. Besides removing a cancerous tumor, surgeons also remove the sentinel lymph node draining the tumor to send to pathology for further analysis. A cancer-free lymph node is a good indicator that the cancer has not spread. Searching for the node can be time-consuming and can also result in tissue damage that fluorescence image-guided surgery can help prevent.
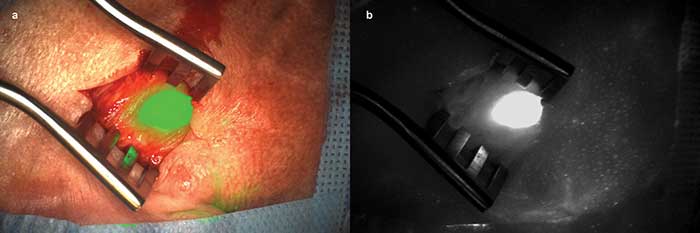
A lymph node is shown before extraction inside the body. The left image (a) shows the color image with the infrared image overlaid in green. The right image (b) shows the accumulated ICG in the lymph node. Courtesy of Quest Medical Imaging.
For this situation, the imaging agent is injected 10 to 20 minutes before visualization, but this time around the tumor tissue, not the bloodstream. The lymph node draining the tumor now drains fluids from the tumor that has taken up the imaging agent. This results in a lymph node structure map where all lymph channels are highlighted between the tumor and the first lymph node. In the infrared region, the excitation light can penetrate the tissue to 8 to 12 mm to excite the imaging agent. The signal emitted by the agent then can be picked up through the tissue by the camera system, in turn showing all locations where the imaging agent is present.
The surgeon can then remove the primary lymph node and the cancer tumor.
It would be a big step forward if it was possible to securely differentiate cancerous tissue from healthy tissue. Various papers1 have shown that this can be done with spectral camera systems based on touch technology looking at cell level. However, the practicality of these applications is specific to certain types of surgery. Brain cancer is one of these, as these are expensive surgeries performed with microscopic imaging systems. However, implementing these types of technologies during the surgical removal of breast cancer would be overly costly and time-consuming. In these situations, it is more practical and less harmful to take away a larger piece of tissue around the tumor margin and/or use spectral analysis to distinguish between cancerous or noncancerous tissue, as has been established in brain cancer trials.
One promising new application of fluorescence image-guided surgery is for the treatment of liver cancer2. Studies have shown that it is possible to visualize liver tumors and metastatic nodes with a contrast agent like ICG. This improves patient outcome because more tumors are identified and removed. All ICG accumulates in the liver before being cleared from the body. But the ICG cannot penetrate the tumor outer wall — instead it builds up around the growth in a ring that shows the tumor margin. This only works when sufficient amounts of ICG are present and/or passing through, which is the case with the liver because ICG is excreted through it. With kidneys, for example, this would not work.
The liver is an organ in which light has very little penetration and as a result only surface tumors can be visualized. During liver surgery, however, a new application is on the horizon that combines visible images, fluorescent images and CT scans to provide the surgeon with a 3D model that shows structures in the liver in real time. This application is still a couple of years out, but the technology is getting ready.
Another promising trend is the move to add fluorescence imaging capabilities to laparoscopic surgery. Laparoscopic surgeries have less impact on the patient, with fewer scars and faster healing time, and since patients generally recover faster, they have a positive effect on hospital costs. The challenge here is to add fluorescence imaging without reducing the quality of the visible image and, at the same time, keeping the smallest possible device for agility. This means creating a small, lightweight camera that can capture high-resolution images at high frame rates.
Furthermore, surgeries started as laparoscopic can be changed to open surgery when complications arise. This puts another requirement on system designs: to have both a laparoscopic capability as well as an open surgery capability. Hospitals do not want the burden of having two separate systems or needing to roll in a new system into an already ongoing surgery.
New optical technologies in multichannel imaging systems have been developed to solve this challenge. The Quest Spectrum imaging system is one such system that can be used for general laparoscopic surgery, but at the same time can add infrared capabilities for both laparoscopic and open surgery. It can sense multiple wavelength channels at exactly the same time along with a color image, HD full resolution at 60 fps. It also has the capability to switch its laparoscopic lens system for an open surgery lens during an active surgery. Furthermore, it has both a color image as well as the infrared image. There are other systems out there as well that have infrared capabilities. Some only provide the infrared image in black and white, or just provide an open lens configuration. Some others only provide a laparoscopic configuration.
The amount of data generated by the system and the processing required to generate high-quality color images is huge. In addition, the necessity of providing multiple infrared images of different optical wavelengths in different colors for different imaging agents requires larger imaging heads. This runs counter to the small imaging system required for laparoscopic surgery. The goal is to provide imaging systems with similar size and shape as current laparoscopic systems but with increased functionality.
This is a huge challenge, but using new imaging sensor technology — including advances in electronic components (components get smaller and smaller), PCB production capabilities (using technologies like buried vias or vias in pads) and newly designed optical prisms prototypes — make this possible. Currently prototypes of next-generation systems allow for five optical channels at the current size of standard laparoscopic systems. This is a huge step in making infrared and, specifically, hyperspectral imaging heads possible, without incurring the increase of size of the optical heads.
Meet the authors
Richard Meester is the CEO of Quest Medical Imaging; email: [email protected]. Sander de Jonge is the technical project lead for Quest’s Spectrum Platform; email: [email protected].
References
1. J. Murphy (Feb./March 2017). Spectroscopy: new uses in surgical suites, laboratories. BioPhotonics, pp. 43-45.
2. P.B.A.A. van Driel et al. (2014). Characterization and evaluation of the Artemis Camera for fluorescence-guided cancer surgery. doi: 10.1007/s11307-014-0799z.
3. M.S. Bradbury et al. (2013). Clinically-translated silica nanoparticles as dual-modality cancer-targeted probes for image-guided surgery and interventions. Integr Biol, Vol. 5, Issue 1, pp. 74-86.
Contrast Agents Evolve
In recent years, various companies have started developing targeted contrast agents. These contrast agents are essentially built of one or more “light bulbs” that are chemically connected to a molecule that only attaches to receptors put out by cancerous cells. The molecule basically sticks to the receptor in the tumor cell. So does the “light bulb” in the contrast agent that is linked to the targeted molecule. From a camera perspective, the process is the same as with the nontargeted contrast agent, except that the agent only stays at the location where tumor cells are present.
The type of administration is different. Rather than being injected directly or 10 to 20 minutes prior to visualization as ICG is, targeted agents need to clear from the circulation system before being imaged. Only the agent that sticks to the tumor cells is visualized. This means that injection time can range from a couple of hours before surgery up to 24 or 36 hours in advance, depending on the agent.
Not every cancer cell expresses the same receptors or “grabbers.” This means that different agents with different chemical constructions have been developed for different cancer types. Furthermore, no human is the same, and people may not respond the same to an agent’s “grabbing” capabilities3. For this reason, and to detect various imaging agents, different agents are made to fluoresce at different wavelengths. This allows multiple agents to be picked up by a single camera system at exactly the same time, discriminating between each. This feature is at the most cutting-edge of intraoperative fluorescent imaging and will be the topic of active research in the future.