The progression of laser-based technology in dentistry has laid the foundation for a cultural paradigm shift from a reactive past to a preventative future.
BEN FISHER AND TIM KILLEEN, ACCESS LASER CO.
Since the late 19th century, the mechanical drill has served as the cornerstone of dentistry. However, as dentists’ responsibilities continue to expand — and after decades of academic research resulting in the advancement of cost-effective, robust, and safe laser technology — a transformation in the dental-care field is arriving. There is an intersection of laser-based instrumentation for imaging, pain management, and disease prevention, where primary operational tools are transitioning the dental profession into the modern paradigm of preventative medicine.
Dentists have traditionally been reluctant to use drills and other tools after identifying white spot lesions — the nascent form of cavities — as limited tools prevented them from intervening because too much healthy tooth would be damaged in the process. Medical lasers for use in dentistry began entering the commercial marketplace in the 1990s. Originally, Nd:YAG lasers were used. Operating at the 1.064-µm wavelength, they remained large and costly, and provided limited soft-tissue capabilities with little to no hard-tissue efficacy. These soft-tissue-only systems improved with the introduction of 10.6-µm CO2 lasers that provided quicker healing times, as well as hemostatic incisions because the wavelength exceeded the average diameter of 7 µm for red blood cells.
Still, these wavelengths only supplanted scalpels. An all-tissue solution was needed; this prompted the introduction of Er:YAG 2.94-µm wavelength lasers. This wavelength’s exceptional absorption in H2O for the first time allowed a laser to adequately address both soft- and hard-tissue dentistry (Figure 1)1. However, the absorption of energy in water to induce a phase change and create microbursts of expanding gas with which to ablate hard tissue left much to be desired in the remaining surface of the tooth.
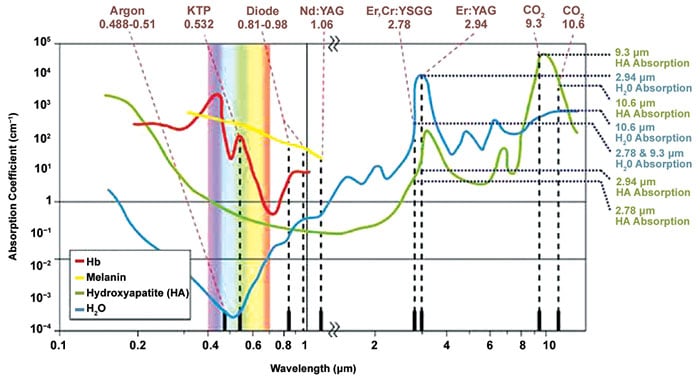
Figure 1. Correlation of material absorption coefficient and laser wavelength; both axes are exponential1. Courtesy of Daniel Fried/University of California, San Francisco.
Dental enamel and dentin (Figure 2) are mostly carbonated-hydroxyapatite by weight, 96 and 85 percent, respectively. For any direct ablation to occur, the laser in use must produce a wavelength with a high absorption coefficient of this mineral compound. In 2005, CO2 lasers — at 9.3 µm with a pulse duration in the microsecond range — were shown to be well suited for the ablation of enamel, dentin, and bone with minimal thermal damage2. By having both a high absorption coefficient and short thermal relaxation time in hydroxyapatite, the DL-500 9.3-µm laser fulfills the requirements of any dental laser — to protect the pulp chamber from increasing by 5.5 oC and avoid damage to the tooth’s blood vessels and nerves. By ablating enamel and dentin through this primary photomechanism rather than water or mechanical methods, the microcracks formed in the underlying healthy enamel and dentin are reduced by over 46 percent3.
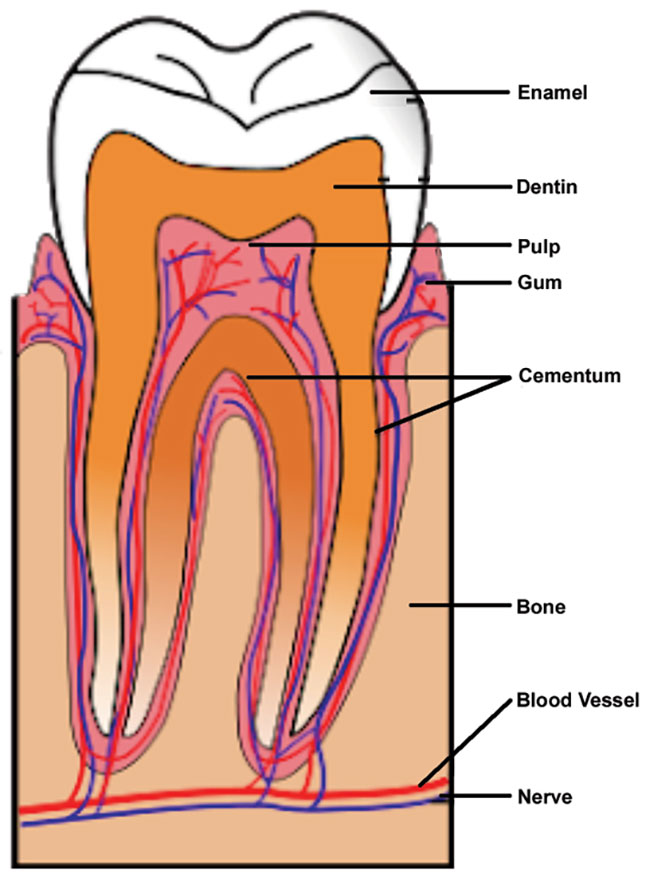
Figure 2. The labeled anatomy of a human tooth. Courtesy of Heath Brantley.
Consistent doctor-patient interaction is a necessary step toward preventative dentistry and is an effective way to enhance dental health. This is sometimes hampered by dental phobia. But newer CO2 lasers are addressing such fears, as they reduce the need for anesthesia by 90 percent and eliminate painful vibrations of standard dental drills. For patients with high sensitivity, another laser solution can be implemented in the form of low-level laser therapy (or photobiomodulation therapy). By bathing targeted areas in 0.6- to 1-µm light, patients have reported analgesic effects, improving cell metabolism and reducing tissue inflammation. With no need to desensitize a patient’s oral cavity and by reducing swelling and bleeding, laser dentistry can implement the multiquadrant approach. This allows the dentist to deal with multiple areas of the mouth in a single visit.
Advancements in imaging technologies have offered dentists the ability to create 3D computer models with detailed accuracy to the micrometer scale of resolution, giving them the confidence to take action during the early stages of white spot lesion formation. To achieve this, NIR lasers generate a point map that provides detail on the water density of the tooth structure.
When demineralization occurs, the less dense cavity has a higher ratio of water than the healthy structure. Exact locations and depths for the proper laser to use to remove the damaged tissue, while preserving the surrounding healthy structure, can be obtained. This is an important distinction, as dentists can easily misidentify a white spot lesion that has been naturally contained when using standard x-ray imaging. With high lesion contrast at 1.88 µm, a dual NIR imaging and 9.3-µm CO2 ablative laser system can be used to selectively remove carious lesions with a mean loss of only 12 µm of sound enamel4. Therefore, the precision enabled by a laser to that of the mechanical drill is multiplied by the additional precision that a computer-guided scanning system provides to the dentist (Figure 3)4.
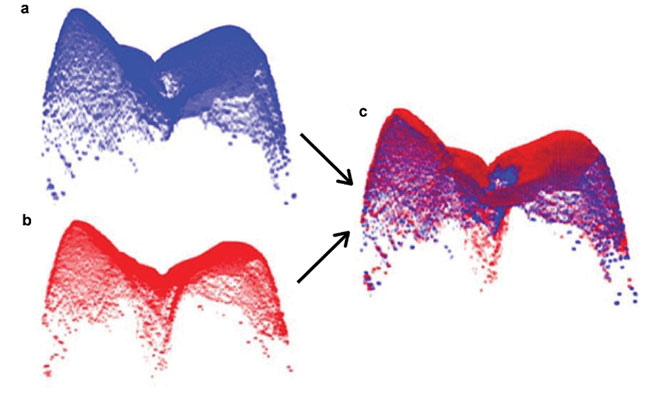
Figure 3. 3D surface imaged with co-polarization optical coherence tomography (CP-OCT). 3D preablation surface (a); 3D post-ablation surface (b); pre-/post-ablation surface 3D registered to determine volume of tissue removed (c). See reference 4. Courtesy of Daniel Fried/University of California, San Francisco.
Beyond simply replacing current tools with their higher precision laser counterparts, the dental workstation of the future will enable a host of new procedures to further provide preventative measures. One such example is the use of the 9.3-µm laser at very low fluences, where instead of ablating the dental hard tissue, the surface temperature is merely increased to release the carbonate bond from hydroxyapatite. This crystallographic change of enamel yields a denser and more acid-resistant layer. The transformation increases the acid-resistant qualities of enamel by as much as
87 percent5.
For patients who already have carious decay in place, the 9.3-µm dental laser provides unique benefits for the preparation of enamel and dentin when placing inlays and onlays. Traditionally, when removing the carious tissue with a mechanical high-speed dental drill, a smear layer is formed that covers the tooth surface with particulate matter; this decreases the strength of the bonding agents. To remove the smear layer and reveal the dentin tubules, to which the applied bonding material needs to attach, an acid etch using 35 percent phosphoric acid is applied. However, this procedure has a side effect: demineralizing the previously healthy structure up to a depth of 20 µm. This can cause a secondary infection after the composite has been set6. Conversely, lasers produce a smooth and clean surface that allows maximal adhesion to the exposed dentin tubules (Figure 4)7.
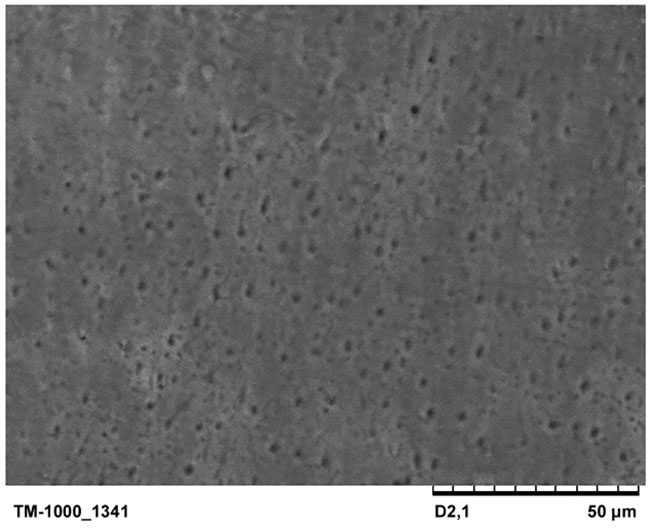
Figure 4. Imaging of the dentin surface using a scanning electron microscope after laser preparation. The holes are dentin tubule openings to which dental resins can fill and create strong bond strengths. See reference 7. Courtesy of Scientific World Journal.
When chronic deterioration requires tooth extraction, the 9.3-µm dental laser also can be of exceptional use in implant preparation. Typically, when a tooth is removed, special care must be taken to ensure that all fibrous material has been cleaned out of the cortical pocket. This can be done with scalpel and scrappers, but only a laser can produce the microholes that puncture the cortical bone and allow blood to flow into the implant site. This greatly increases the attachment rate of osteoblasts to the titanium threads of an implant screw, ensuring significantly higher success rates while lowering infection probabilities.
Advancements in imaging technologies have given dentists the ability to create 3D computer models with accuracy to the micrometer scale of resolution, giving them the confidence to take action during early-stage lesion formation.
The germicidal benefits of lasers in prosthodontics are becoming increasingly apparent as global populations continue to age faster and live longer. Subsequently, there is a correlative rise in tooth loss. This issue is further exacerbated by the prevalence of Type 2 diabetes throughout the U.S. To provide those with tooth loss an increased standard of living, prosthodontists use titanium screws to secure bridges and crowns. However, 14.4 percent of patients experience peri-implantitis, a destructive inflammatory process affecting the soft and hard tissues surrounding dental implants, resulting in decreased implant attachment strength and loss of bone density8.
When recently machined titanium is exposed to air, the metal oxidizes and its surface transforms, making it no longer hydrophilic. This encourages fibrous attachment and osteoblast stimulation to a hydrophobic surface, which creates irritation and allows infectious diseases to take root. While many dentists will see patients who have received care from other practices and cannot control the conditions in which the implant was initially placed, they can perform either open flap or closed flap decontamination of the screw surfaces with a 9.3-µm laser. In clinical studies, a CO2 laser demonstrated positive results when decontaminating implant surfaces and bone filling with autogenous bone or bone-grafting materials; this leads to long-term success of the oral implants9.
With all of these various benefits ranging from pain management, all-tissue surgery, and preventative and postoperative care to 3D milling of custom implants, an all-in-one dental workstation of the future only requires one more integration to fully maximize its potential — the final step needed to pull it all together is a software package that uses an NIR imaging system to map a patient’s teeth and identify which areas require action. This linchpin could be a midlevel AI interface that is able to operate semi-autonomously with only critical decisions and junctures made by a dentist, similar to modern commercial airplanes that are largely automated with only specific stretches of a flight plan needing human intervention.
The future of dentistry should bring a more streamlined process of automation in which dentists are present to provide high-level strategic insight while allowing a machine to implement the traditional tasks of providing tissue interaction. By utilizing the aforementioned NIR imaging, a precise point map can be created, denoting areas of interaction on a scale of just tens of microns, which is several orders of magnitude more precise than traditional caries removal.
Achieving this and realizing the potential of preventative dentistry will require a groundswell stemming from both academia and professional organizations. For practicing dentists, these benefits will only be accessible once insurance companies modernize their billing codes to reflect the fiscal and societal advantages that these revolutionary devices offer.
The dental workstation that utilizes a 9.3-µm dental laser enables safe, efficient, patient-friendly visits to the dental office. They need to also be widely available, compact, and intuitive for dentists to learn.
Providing short pulse widths of 5 to 25 µs with pulse energies of 1 to 15 mJ allows efficient and safe ablation of hard tissue while simultaneously providing exceptional soft tissue work. Such a light source will be the heart of next-generation workstations and help to redefine dental visits from dreaded chores to quick, painless checkups to keep teeth healthy and clean.
Meet the authors
Ben Fisher is an applications and sales engineer at Access Laser. He has a master’s degree in physics from the University of Washington; email: [email protected].
Tim Killeen is sales and marketing manager at Access Laser. He has a bachelor’s degree in electrical engineering; email: [email protected].
References
1. J.D.B. Featherstone et al. (2001). Fundamental interactions of lasers with dental hard tissues. Medical Laser Application, Vol. 16, Issue 3, pp. 181-194.
2. D. Fried (2005). Laser processing of dental hard tissues. Proc SPIE, Vol. 5713, pp. 259-269.
3. L.H. Maung et al. (2010). Near-IR imaging of thermal changes in enamel during laser ablation. Proc SPIE, Vol. 7549.
4. K. Chan et al. (2018). Selective ablation of carious lesions using an integrated multispectral NIR imaging system and a novel 9.3-µm CO2 laser. Proc SPIE, Vol. 10473.
5. P. Rechmann et al. (2011). Caries inhibition in vital teeth using 9.6-mm CO2 laser irradiation. J Biomed Optics, Vol. 16, Issue 7.
6. A. Moritz (2018). Latest scientific results in dental hard tissue preparation. Academy of Laser Dentistry Conference.
7. M.A. Al-maliky et al. (2014). The effects of CO2 laser with or without nanohydroxyapatite paste in the occlusion of dentinal tubules. Sci World J, Vol. 2014.
8. G. Romanos et al. (2009). The use of CO2 laser in the treatment of peri-implantitis. Photomed Laser Surg, Vol. 27, Issue 3.
9. G. Romanos et al. (2008). Regenerative therapy of deep peri-implant infrabony defects after CO2 laser implant surface decontamination. Int J Periodontics Restorative Dent, Vol. 28, Issue 3, pp. 245–255.