Adaptive optics on the Giant Magellan Telescope sense optical aberrations caused by atmospheric turbulence and correct them in real time using a deformable mirror.
ANTONIN BOUCHEZ, GIANT MAGELLAN TELESCOPE ORGANIZATION (GMTO CORP.)
Under ideal circumstances, the resolution of an optical system is limited by the diffraction of light waves. This diffraction limit, the maximum resolution a telescope can achieve, improves linearly with the telescope’s aperture. However, the view of the universe provided by ground-based telescopes is typically limited not by diffraction, but by atmospheric blurring due to temperature inhomogeneities and turbulence in the Earth’s atmosphere. Even the best astronomical sites in the world, located on remote mountaintops with particularly stable air, degrade diffraction-limited images to 0.6 to 0.7 arcseconds. While this is approximately 100 times finer than the naked eye can achieve, it is no better than the diffraction limit of a modest backyard telescope.
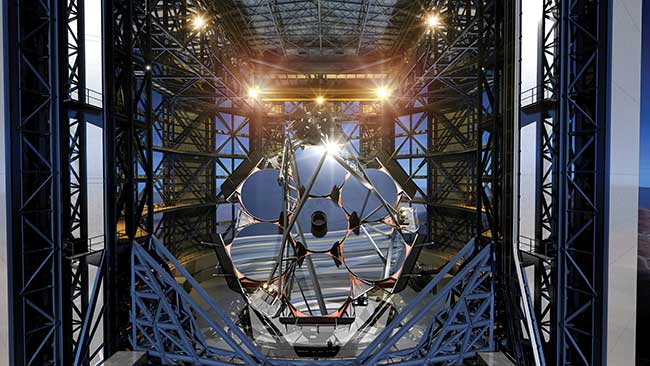
The Giant Magellan Telescope is a segmented mirror telescope that employs seven of today’s largest stiff monolith mirrors as segments. Six off-axis 8.4-meter or 27-foot segments surround a central on-axis segment, forming a single optical surface 24.5 meters, or 80 feet, in diameter. Courtesy of Giant Magellan Telescope — GMTO Corp.
Space telescopes do not suffer from the limitations imposed by atmospheric turbulence. The 2.4-m-diameter Hubble Space Telescope can produce images with detail as fine as 0.04 arcseconds in visible light. The 6.5-m-diameter James Webb Space Telescope, due to be launched in 2018, will have nearly three times higher resolution in the infrared, but its mirrors are not specified to achieve the diffraction limit in the visible.
Adaptive optics
Adaptive optics (AO) allows ground-based telescopes to approach the resolution of comparably sized space telescopes. Adaptive optics systems sense the optical aberrations caused by atmospheric turbulence, and correct them in real time using a deformable mirror. The deformable mirror must be adjusted hundreds of times per second to keep up with the evolving turbulence. Most large ground-based observatories are now equipped with AO systems to feed their narrow field-of-view infrared instruments, improving their resolution by over a factor of 10. These instruments have made spectacular discoveries, including the first images of planets around other stars, storms on Saturn’s moon Titan, and the discovery of a super-massive black hole at the center of our galaxy.
The Giant Magellan Telescope (GMT) is a 24.5-m-diameter telescope being built at Las Campanas Observatory in Chile by a global consortium of universities and research institutions. It is one of three “extremely large telescope” (ELT) projects currently underway, all of which plan to start initial science operations in 2022 to 2024.
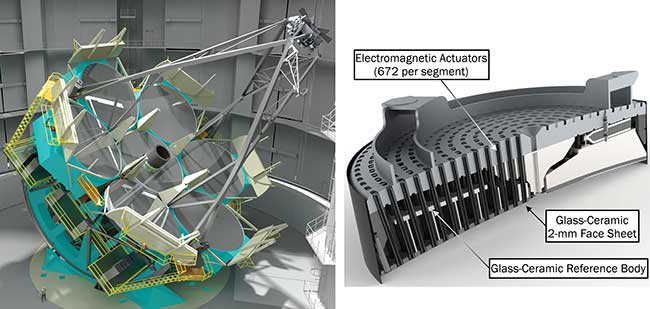
Rendering of the Giant Magellan Telescope (left) and a cross section of an adaptive secondary mirror segment (right). Courtesy of Giant Magellan Telescope — GMTO Corp.
The GMT has a segmented primary mirror composed of seven 8.4-m-diameter glass segments with a common focus. These giant mirror segments are currently being manufactured by the Richard F. Caris Mirror Lab at the University of Arizona, one of the GMT founding institutions. Unlike most current-generation telescopes, GMT incorporates an adaptive secondary mirror, thus providing atmospheric turbulence correction to every instrument on the telescope. This design was pioneered at the Large Binocular Telescope on Mount Graham in southeastern Arizona. The GMT adaptive secondary mirror is segmented, with seven 1-m-diameter circular segments matching those of the primary mirror. Each of these deformable mirror segments has 672 electromagnetic actuators that levitate and bend a 2-mm-thin glass face sheet with permanent magnets bonded to its back. The resulting adaptive mirror can be updated 2,000 times per second, providing precise atmospheric correction with no need for additional optics ahead of the scientific instruments.
A single deformable mirror can only perfectly correct the optical aberrations caused by atmospheric turbulence along a single line of sight through the atmosphere. Alternately, the deformable mirror can be commanded to correct the average turbulence over an extended field of view. This trade-off allows the adaptive optics correction to be tuned to the observations being performed. Bright guide stars near the scientific target of interest are required to measure the atmospheric aberrations with sufficient speed. If these are not available, then artificial guide stars generated by the backscatter of laser light in the upper atmosphere can be used. These considerations have led to the development of a wide variety of AO control techniques, including natural guide star AO (NGAO), ground-layer AO (GLAO) and laser tomography AO (LTAO).
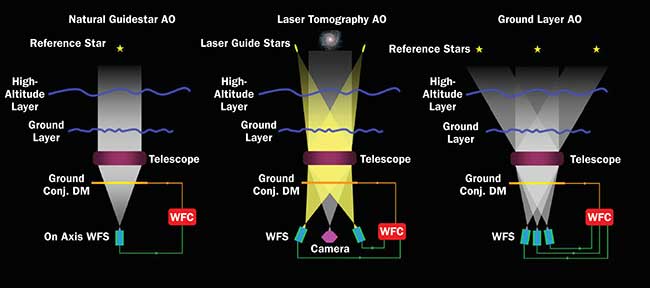
Schematic representation of three possible adaptive optics control techniques. In each case the output of wavefront sensors (WFS) is analyzed by a wavefront control computer (WFC) to update a deformable mirror (DM). The wavefront sensors can be fed by either real reference stars or back-scattered laser light. Courtesy of Enrico Marchetti, European Southern Observatory.
Direct detection of exoplanets
Natural guidestar AO is the simplest form of AO employed on the GMT, used when a bright guide star is available near the scientific target. It provides the highest resolution and highest contrast images, over a narrow field of view. NGAO is particularly well-suited to the study of extrasolar planets orbiting nearby stars, since these stars provide an ideal reference. Over 3,000 extrasolar planets have now been identified, nearly all using indirect detection techniques such as monitoring the Doppler shift of the parent star or the photometric signature of a planetary transit across the parent star. The planets themselves are lost in the glare of the parent star, which is typically 104 to 108 times brighter and just 0.1 to 1.0 arcseconds away. Adaptive optics provides the only method to directly image these planets and analyze their reflected and emitted light.
The natural guide star AO mode on the GMT will be able to reach planets up to 107 times fainter than the parent star in the near-infrared, at just 0.1 arcseconds from the star. This is sufficient to image Saturn-mass planets around many stars in the solar neighborhood, and perhaps a small number of “super-Earths,” rocky planets several times the Earth’s diameter, around the nearest stars. Spectral analysis of the light from these planets can yield vastly more information than the few orbital parameters that the indirect detection methods provide. It could even provide the first signatures of life of these planets, if biomarkers such as the simultaneous presence of ozone and methane are detected. This goal is beyond the reach of present-day telescopes, but well within the capabilities of the coming ELTs.
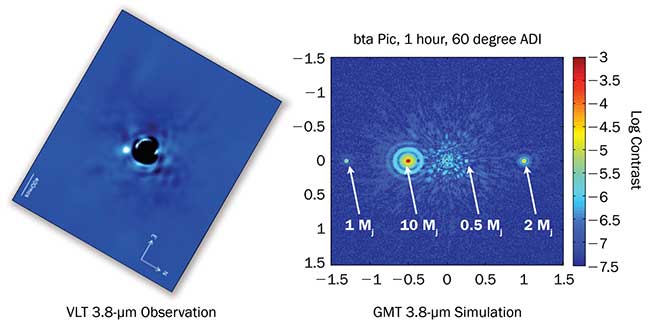
Simulated Giant Magellan Telescope (GMT) image of a hypothetical planetary system orbiting Beta Pictoris, located 63 light years from Earth (right) compared to real observations by the European Southern Observatory’s Very Large Telescope, which discovered a 10 Jupiter-mass planet1 (left). GMT will be capable of detecting planets as small as Saturn in this system. Courtesy of Phil Hinz, University of Arizona.
Ground-layer AO improves a telescope’s image quality less dramatically, but over a far wider field of view. This is done by correcting only the portion of the turbulence located near the ground (typically less than 500 m above the telescope), through which lines of sight overlap. The resolution improvement provided is approximately a factor of 2, reducing typical image size to 0.2 to 0.3 arcseconds over fields of view as large as 20 arcminutes (two-thirds the apparent size of the full moon). This is usually sufficient resolution for studies of very distant galaxies, which have low surface brightness and little structure at finer scales. GLAO will therefore allow the ELTs to study the most distant galaxies in large numbers, with unprecedented sensitivity.
Laser guide stars
One of the most significant astronomical findings enabled by the last generation of 8- to 10-m telescopes was the discovery of a super-massive black hole at the center of the Milky Way2. This black hole contains 4 million times the mass of our sun, and dominates the dynamics of the stars in the inner part of the Milky Way galaxy. Only with adaptive optics can the thousands of stars orbiting the central black hole be resolved and their orbital motions measured. These orbits reveal the mass of the central black hole, Sagittarius A*, and also the history of star formation and destruction in its vicinity. Mid-infrared flares emanating from an accretion disk around the black hole have been imaged with adaptive optics, providing evidence of its continual growth3.
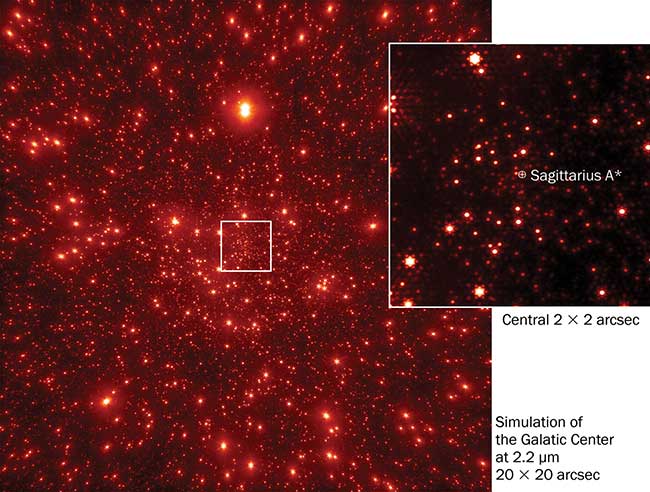
Simulated Giant Magellan Telescope (GMT) laser tomography adaptive optics image of the region surrounding Sagittarius A*, the super-massive black hole at the center of the Milky Way. Known star positions were provided by Tuan Do and the UCLA Galactic Center Group. Courtesy of Giant Magellan Telescope – GMTO Corp.
While the millions of stars in the galactic bulge generally provide excellent AO guide stars, Sagittarius A* is hidden behind thick clouds of gas and dust, completely obscuring the region in the visible wavelengths at which wavefront sensors typically operate. Laser guide stars must therefore be used to provide the atmospheric turbulence measurements that enable adaptive optics. Single-laser guide star systems are now deployed at most major observatories, but the ELTs require more sophisticated systems with multiple lasers to sample the wide column of atmosphere through which the telescope is pointing. The GMT system will use six lasers whose measurements will be combined tomographically to derive the optimal adaptive secondary mirror commands in the direction of the scientific target. The resulting resolution of 0.02 arcseconds is sufficient to track the motion of the innermost stars over periods of weeks, and will allow the effects of general relativity to be revealed as stars pass within light hours of the Sagittarius A* event horizon.
Astronomy is a technology-driven science, with unexpected discoveries made with each new generation of larger and more powerful telescopes. The next generation of ground-based ELTs will be revolutionary due to their high spatial resolution — unrivaled by even the largest envisioned space telescopes. The GMT will benefit from a resolution improvement of a factor of 2.5 over currently existing telescopes, enabling the characterization of super-Earths orbiting nearby stars, the study of the most distant galaxies in the universe, and the detection of new physical phenomena in the vicinity of super-massive black holes. However, its most exciting discoveries cannot yet be predicted.
Meet the author
Antonin Bouchez is GMTO’s Wavefront Sensing and Control Group Lead, and is responsible for designing and implementing the adaptive optics systems for the Giant Magellan Telescope; email: [email protected].
References
1. A.-M. Lagrange and M. Bonnefoy, et al. (2010). A giant planet imaged in the disk of the young star ß Pictoris. Science, Vol. 329, p. 57-59.
2. A.M. Ghez and B.L. Klein, et al. (1998). High proper motion stars in the vicinity of Sgr A*: Evidence for a supermassive black hole at the center of our galaxy. Astrophysics, Vol. 509, pp. 678-686.
3. R. Genzel and R. Schödel et al. (2003). Near-infrared flares from accreting gas around the supermassive black hole at the Galactic Centre. Nature, Vol. 425, pp. 934-937.