Extreme Laser Stability Key to aLIGO and VIRGO Successes
An actively stabilized nonplanar ring oscillator (NPRO) is at the heart of aLIGO, VIRGO, and all gravity-wave detection systems around the world.
MANTAS BUTKUS, VOLKER LEONHARDT, AND MARCO ARRIGONI, COHERENT
By any measure, gravitational-wave detection and analysis has proved to be a spectacular success for “big science.” It also represents one of the singular achievements of the photonics community, pushing our industry’s technology orders of magnitude beyond the stability and sensitivity limits needed in any previous application.
Conceptually, LIGO is simple: It uses laser interferometry to measure small changes in the distance between three optics as minor transient ripples in space-time pass by. But nothing about its practical implementation is simple, since it requires measuring space-time oscillations as small as 1 part in 1022. That’s like measuring the distance to the moon to within 10 millionths of the width of a human hair!
The extreme long-path laser interferometry that LIGO utilizes requires a highly stable laser source. It also requires high power (~100 kW) so the quantum noise limit of the laser does not overwhelm the signal. Fortunately, each arm of the interferometer acts as a cavity. So with an input of ~160 W of amplified laser power, this results in very high (~100 kW) circulating power.
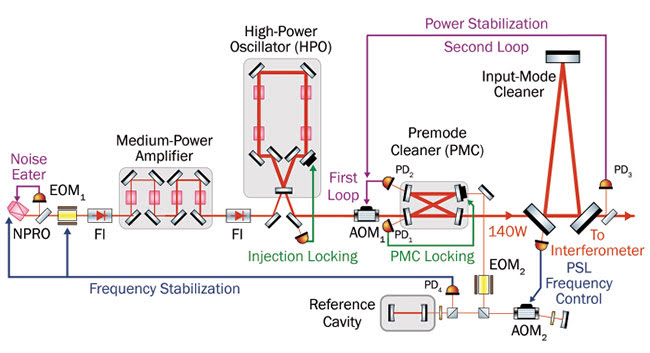
Schematic layout of the prestabilized laser (PSL) system developed for LIGO, showing the key components and feedback stabilization loops. NPRO: nonplanar ring oscillator; PMC: premode cleaner. Adapted from reference 1.
Courtesy of Caltech.
All gravitational-wave interferometers in use and under development employ an NPRO operating at 1064 nm as the initial laser oscillator, and they all use the Coherent Mephisto, based on a design specifically developed for this purpose. As described in the August 2018 issue of this magazine (see “Quiet Lasers, Cool Science”), a single diode-pumped Nd crystal acts as both the gain medium and a miniature cavity in an NPRO. This is inherently the most stable type of laser oscillator yet developed. The 2 W of output power is then stepped up to the requisite level by several stages of amplification.
Both the NPRO oscillator and the amplifier stages have to be actively stabilized. The Mephisto includes three inputs for this purpose: pump diode current control for short-term power stabilization, piezoelectric squeezing of the NPRO crystal for frequency (and phase) control, and temperature control for long-term frequency stabilization.
The fine details of the amplification stages and stabilization schemes are slightly different in each interferometer. But a brief look at the prestabilized laser (PSL) arrangement used in the aLIGO (advanced LIGO) gravity wave interferometers at Hanford, Wash., and Livingston, La., reveals important common and fundamental aspects (see schematic at right). The aLIGO PSL system encompasses the NPRO, followed by a medium-power amplifier whose output is then used to injection-lock a high-power oscillator in a ring configuration. This PSL setup is capable of reaching 220 W of output.1
There are three major loops through which the PSL output is stabilized.
Mode Stabilization. The NPRO is a laser that is already configured for operation at a single longitudinal mode. In addition, the PSL incorporates a feedback loop to suppress any higher-order transverse mode components. This is achieved by the use of a passive ring resonator called the premode cleaner (PMC); here the so-called Gouy phase shift acts to block transmission of unwanted modes. The PMC resonator is in a bow-tie configuration with the two total reflectors having a reflectivity of >99.995 percent to minimize loss for the resonator.
Frequency Stabilization. Clearly, frequency stabilization is critical in aLIGO. During PSL development and qualification, the NPRO output is locked to a reference cavity using the piezo input. In the final implementation, the output is locked to the arm cavities in the interferometer itself. To avoid any possibility of frequency/amplitude noise confusion, the Pound-Drever-Hall (PDH) locking technique was chosen for this task because PDH locking produces a signal zero, which is independent of laser amplitude fluctuations.
Power Stabilization. The main source of power noise is the high-power oscillator (HPO), not the NPRO. This noise is addressed by the clever use of two nested loops: one based on a signal from the output of the PMC (i.e., just before the HPO) and one from a photodetector (PD3) just prior to the interferometer itself. This arrangement meets the impressive power stability of 2 × 10-9 Hz-1/2 at 10 Hz at the input of the LIGO interferometer.
Successful gravitational wave detection represents a triumph for lasers and other photonics technologies, shining a light on one of the most complex questions in astronomy.
Meet the authors
Mantas Butkus, Ph.D., is a product line manager at Coherent Inc. for multiple laser products for scientific and industrial markets; email: [email protected].
Volker Leonhardt is a product support engineer at Coherent Inc. and is responsible for the Mephisto product line; email: [email protected].
Marco Arrigoni is a director of strategic marketing at Coherent Inc. He covers the scientific research markets; email: [email protected].
Reference
1. P. Kwee et al. (2012). Stabilized high-power laser system for the gravitational wave detector advanced LIGO. Opt Exp, Vol. 20, Issue 10, pp. 10617-10634.
/Buyers_Guide/Coherent_Inc/c2706
Published: September 2018
LIGOgravitational waveGWaLIGOAdvanced LIGOLaser Interferometer Gravitational-Wave Observatorychirpblack holesVirgoGW170817gamma-ray burstgalaxy NGC4993sGRBgravitonsinterferometer CaltechKavli InstituteLISAGariLynn BillingsleyFeatures