The bistability and unique polarization of ferroelectric liquid crystal displays position them as front-runners for microdisplay technology.
Daniel Krueerke and Ruppel Joshi, CRL Opto Ltd.
Microdisplays are miniature, high-resolution displays that typically measure 1 cm diagonally and whose use requires magnifying optics. These displays can be transmissive, reflective or emissive. The transmissive type based on twisted nematic liquid crystals using the waveguide effect are well-established. They use active-matrix liquid crystals to modify the state of polarization of the light propagating through the assembly. Reflective devices, on the other hand, use liquid crystal on silicon (LCOS) to modulate the state of polarization of the reflected light or to mechanically deflect the incident light via backreflection. Emissive displays generate light and therefore do not require an external light source.
Most reflective LCOS microdisplays work with nematic liquid crystals, but ferroelectric liquid crystal is a viable alternative. The key advantages of the latter are very fast switching times (100 μs) and their inherent bistable nature. Bistable displays are matrix-controlled and store information at their pixel configuration. An element needs to be addressed only once to make sure that it is on or off. Bistability is not easily achieved with nematic liquid crystal because bistable nematic displays with reasonable performance require sophisticated, nanostructured alignment layer topographies to introduce two stable states.
The waveguide effect, which guides the polarization plane of light through the liquid crystal layer, is not applicable in reflective devices without a backplane polarizer, or complicated cell gap structure. The light would propagate along the same spiral staircase down to the mirror as on the return journey to the cell window. Therefore, there would be zero net change for a polarized input light beam when it traverses the cell.
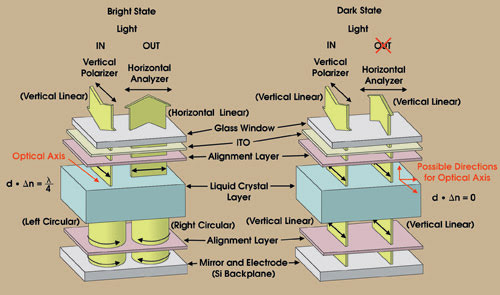
Figure 1. This schematic illustrates the optical principle of a binary switchable liquid crystal display pixel in reflective mode. The liquid crystal birefringence is used for phase modulation and takes into consideration the fact that circular polarized light changes during normal reflection.
Reflective LCOS devices are purely phase modulators using the birefringence (Δn) of a liquid crystal layer of defined thickness (d) for sufficient retardation (d · Δn). The net λ/2 effect is achieved by adjusting the “bright” state of the single-passed liquid crystal layer for λ/4 conditions; i.e., d · Δn = λ/4, at a suitable wavelength (Figure 1). The LCOS device consists of an open silicon memory chip, a transparent opposite electrode and the liquid crystal layer in between, defined by suitable surface anchoring.
Ferroelectric LCOS
The various liquid crystal phases, usually formed by rod-shaped organic molecules, differ in their structure and therefore in their physical properties. The ferroelectric type is soft, like honey, and its polarization is unique. In the surface-stabilized configuration, its molecular order can be profitably influenced by reversing an electric field applied along the cell normal, a process that is not possible with ordinary nematic liquid crystals.
While commercial nematic liquid crystals rely on elastic relaxation forces for one of the switching states, a ferroelectric liquid crystal cell is actively driven into both states. The switching process is characterized by an in-plane rotation of the optical axis. This process typically takes about 10 to100 μs, which is approximately three orders of magnitude faster than the ordinary switching times achievable with nematic crystals. Additionally, the in-plane switching of the optical axis in the ferroelectric crystal provides excellent viewing angle characteristics (Figure 2).
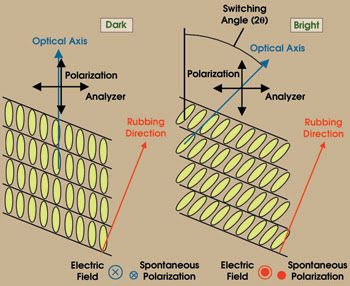
Figure 2. The switching process in a ferroelectric liquid crystal is characterized by an in-plane rotation of the optical axis. This process typically takes 10 to 100 μs, much faster than the switching times achievable with nematic crystals.
Within the ferroelectric smectic C* phase (the * refers to the chiral nature), the molecules are arranged in a layered geometry. They are tilted with respect to the layer normal, which is defined by the alignment direction of the cell (e.g., rubbing direction). The unique property is the coupling between the collective molecular tilt and a spontaneous polarization perpendicular to the plane of the tilt angle. Reversing the direction of spontaneous polarization by the application of an external electric field flips all molecules to the other side, away from the alignment direction (rubbing). In the dark state, the molecules are aligned with the polarization of the incident light; its polarization remains unchanged and cannot pass the analyzer.
True-color projection systems based on nematic LCOS microdisplays are configured with three color channels, for red, green and blue (RGB) information. This requires three microdisplays illuminated with RGB light for each display, and a sophisticated optic for splitting and recombining the three color beams.
Ferroelectric LCOS microdisplays, on the other hand, are fast and cheap, and they can easily be scaled up to high resolution. Therefore, they are destined for operating in a single-channel, full-color, time-sequential mode. Here the information for RGB is flashed onto the same display sequentially by continuous illumination through a spinning color wheel or by LED illumination. Above a certain frequency, the human visual system fuses the short flashing color subimages into full color.
Lighting with LEDs
Although three-channel systems place more light on a screen, they are expensive and require complex alignment mechanics. Achieving both light and resolution at low cost is difficult unless we combine more intelligent illumination techniques with a single-channel ferroelectric LCOS display.
The use of modern ultrabright LEDs for projection offers major advantages. First, pulsed LEDs are more efficient than continuously driven ones, so the effective light throughput of the ferroelectric LCOS panel is higher than in comparable continuous illumination. Second, this type of illumination allows us to split the three color frames into small bit portions and to mix them, overcoming the problems of color breakup and flicker. This sometimes comes from color-field sequential systems with continuous illumination, in which the RGB frames exist in solid blocks. Third, the reliability and lifetime of monolithically fabricated components, such as LEDs and silicon backplanes, far exceeds the standard lifetime of projection lamps to the extent that the overall system reliability of this type of projector becomes that of standard consumer electronic products.
Turning up the light
The evolution of brightness and price for LEDs is progressing in a Moore’s law fashion. Brightness per unit has been increasing at a rate of 30 times per decade. Similarly, the cost per unit of brightness has been decreasing at a rate of 10 times per decade, to about 6 cents per lumen in 2000. Current LED technology would enable microprojector products for instrumentation and, in the near future, small- to medium-size rear-projection TV products. One way of overcoming brightness problems with current LEDs is to couple larger LED arrays and concentrate their light output onto the display with waveguides.
Drive schemes for all liquid crystals must maintain a DC voltage balance to avoid electrochemical degradation processes. Ferroelectric LCOS devices switch to the polarity of the applied field, making DC balance more difficult. In the most conventional method of addressing these devices, referred to as direct-drive, a positive image is written to the device and is followed by a negative image, written using complementary addressing signals. The direct-drive approach is well-suited to applications where the illumination can be pulsed.
In more conventional arc-lamp-based projection systems, it is more difficult to pulse the illumination. The negative image that is a consequence of the direct-drive scheme must therefore be discarded (causing a 50 percent loss in efficiency) or inverted by other optical elements. In some applications, an additional liquid crystal compensation cell can be used to invert the negative image shown on the display, enabling continuous illumination, which helps increase light throughput. In practice, however, this usually compromises the efficiency or performance of the system.
The need for an inverse image can be overcome by using the bistability of the ferroelectric liquid crystal. In these pulse-mode schemes, a short pulse switches the liquid crystal into the desired state. The bistability of the material maintains this state for the required time, and at the end of the period, a DC-balancing inverse pulse is applied. In this way, an inverse image is no longer needed, and the display can be continually illuminated.
The real world
Applications for microdisplays generally can be divided into three categories: direct view, such as head-mounted displays, viewfinders (Figure 3) and simulation binoculars; projection, such as head-up displays and rear and front projection; and nondisplay, such as printing, holography, optical correlation, pulse shaping and telecommunications.
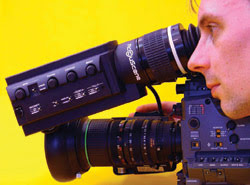
Figure 3. The Accuscene 1280 × 1024 microdisplay-based viewfinder, developed for high-definition cameras, provides a full-color, flicker-free image.
Most imaging applications that integrate microdisplays based on liquid crystal technology use polarized light. Using one state of polarization can result in an efficiency loss of approximately 50 percent. However, this has been addressed by some of the polarization-conversion techniques available today.
The ferroelectric LCOS microdisplay from CRL Opto Ltd. of Dalgety Bay, UK, is an example of an all-digital device driven by application-specific integrated circuits that support multiple video formats, including high-definition TV. These microdisplays can provide high performance at relatively low cost. High-brightness, high-contrast super-extended graphics array (1280 × 1024 pixels) resolution images can be displayed free from flicker, color breakup and image sticking at 120 Hz and 24-bit color (Figure 4).
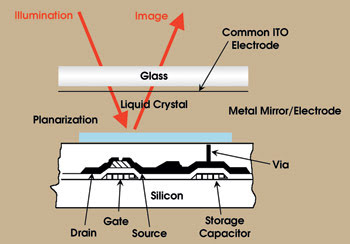
Figure 4. The fast response time of a ferroelectric LCOS microdisplay (typically less than 50 μs) allows color-sequential illumination techniques to generate color images from a single microdisplay. Gray scale and color are achieved with temporal techniques that exploit the fast switching speed and digital characteristics of the device.
For efficient use in systems with continuous illumination, such as that provided by projection engines, the display is best operated in a bistable mode. The exploitation of its bistable nature requires careful consideration of the relevant interactions between alignment layer and liquid crystal, and the processing tolerances are consequently higher. Photoalignment and the evaporation of inorganic alignment layers are noncontact techniques currently used to enable a bistable production display.
Meet the authors
Daniel Krueerke is principal liquid crystal engineer at CRL Opto Ltd. in Dalgety Bay, UK; e-mail: [email protected].
Ruppel Joshi is marketing manager for CRL Opto; e-mail: [email protected].