Already capable of delivering tens of watts, a novel optical fiber offers the possibility of minimally invasive surgery using CO2 lasers.
Charalambos Anastassiou, Gregor Dellemann, Ori Weisberg and Uri Kolodny, OmniGuide Communications Inc.
An innovative class of optical fibers was developed several years ago at Massachusetts Institute of Technology in Cambridge, based on 1998 work with photonic bandgap reflectors, a new type of dielectric mirror that can reflect light and that displays extremely low losses.1 Unlike other dielectric mirrors, which reflect light only for a very narrow angle of incidence at a specific wavelength, photonic bandgap reflectors are capable of very high reflectance for any angle of incidence and for a large band of wavelengths.
This is of particular interest for the fiber beam delivery of mid-infrared radiation from CO2 lasers. Because of their desirable properties for cutting tissue, these lasers are widely used in surgical and dermatological procedures. However, delivering the beam to the patient requires an articulated arm and a handpiece of considerable size. There is no optical fiber on the market that can deliver a CO2 laser beam through a flexible endoscope. This restriction precludes using these lasers in minimally invasive surgical procedures.
The availability of optical fibers for shorter wavelengths has enabled other lasers — such as Nd:YAG and diode — to gain in the medical laser market. The advantages of fiber optic beam delivery outweigh the disadvantages of less-desirable tissue-cutting properties in a broad range of medical applications.
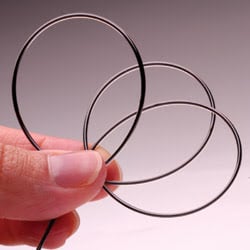
Recent fibers made of a highly flexible polymer achieve loss levels as low as 10 percent per meter.
The new fibers have the potential to change the picture dramatically by enabling flexible CO2 laser beam delivery. In 2002, an MIT group published the first results on such transmission.2 Since then, OmniGuide Communications Inc. has refined the technology, improving the fiber’s performance and reliability. Its recent fibers are made of a highly flexible polymer and achieve loss levels as low as 10 percent per meter.
From reflector to fiber
The principle of a photonic bandgap reflector is straightforward. Just as the periodicity in a semiconductor crystal creates a bandgap through which electrons cannot propagate, the periodicity of the reflector creates a photonic bandgap through which photons cannot propagate. And because the photons cannot propagate into the material, they have no choice but to be reflected.
Recent work has focused on turning these omnidirectional photonic bandgap reflectors into fibers. The concept is simple: Take the mirror and wrap it around a hollow core. The result is a tube that is highly reflective on the inside. If manufactured from glasses and polymers with the right thermomechanical properties, this tube can be heated to the proper degree of softness and drawn into very long lengths of optical fiber.
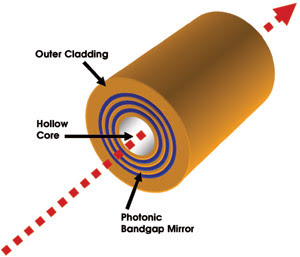
Novel fibers constructed of Å photonic bandgap reflectors enable flexible CO2 laser beam delivery. The photonic bandgap reflector consists of alternating concentric layers of optical materials with different indices of refraction. The choice of materials and the layer thicknesses determine the fiber’s transmission properties.
The optical properties of the photonic bandgap reflector that confines the radiation to the hollow core determine the optical properties of the fibers. The properties of the reflector are to a large extent determined by its structure alone. The reflector consists of alternating concentric layers of optical materials that have two indices of refraction. By selecting the proper materials (and thus adjusting the indices of refraction) and by carefully choosing the layer thicknesses, one can alter the fiber’s transmission properties. In particular, one can change the position of the reflected band of wavelengths in the optical spectrum by scaling layer thicknesses: Thicker layers result in longer transmission wavelengths, and thinner layers result in shorter ones.
The challenge in manufacturing these hollow-core photonic bandgap fibers is the selection and synthesis of the mirror materials. Although the materials must exhibit very similar thermomechanical properties, they also must have very dissimilar optical properties. Once such a material system is identified, however, fibers can be manufactured in a standard preform-drawing process. Using this technique, OmniGuide produces kilometers of fibers every week.
In vitro testing
The fibers have been equipped with standardized connectors, which enable quick fiber replacement without requiring optical realignment. The connector incorporates a metal aperture that protects the highly absorbing edge of the fiber mirror and jacket from laser radiation and that ensures that all incoming radiation is launched into the hollow core of the fiber. The hole in the aperture is left open to allow for gas flow into the fiber. Such a fiber delivery system has the potential to be quite economical. Consequently, the fibers may be designed to be disposable supplies that require neither special care nor sterilization after a medical procedure.
In vitro performance data have been gathered with a prototype of a commercial fiber-delivered CO2 laser system built around a standard ESC Sharplan Medical Systems 40C medical laser. Because the laser does not come equipped with an FC connector, OmniGuide designed an adapter to replace the articulated arm of the laser and to focus the laser beam on the connector’s aperture. The adapter also provides a port for gas flow into the fiber, which keeps debris off of the fiber’s distal end and provides a cooling mechanism.
At the distal end, a protruding metal sleeve protects the end face from direct contact with the tissue. In combination with nitrogen or argon gas flow through the core of the fiber, this sleeve allows continuous contact with the tissue without damaging the fiber. The outside diameter of both the fiber and the distal end sleeve fits within the 2-mm working channel of a flexible endoscope.
The system design demonstrates the feasibility of retrofitting a CO2 laser system with fiber beam-delivery capability. Medical practitioners interested in the technology will not need to buy a new CO2 laser system; they need only upgrade their existing laser.
Using this setup, the fibers were tested at Wellman Labs of Photomedicine at Massachusetts General Hospital in Boston. Histology data from tissue-cutting experiments on pigskin revealed equal or better cut quality for the fiber-delivered beam, compared with beams from current, articulated-arm delivery systems. Stable operating conditions were achieved at input power levels as high as 16 W (CW operation) and 20 W (pulsed operation). Although current development work focuses on increasing the power-carrying capacity of the fiber, these performance levels already are of interest for laryngological applications. The first in vitro tests on a pig larynx have been conducted, and live animal experiments will follow.
New approach, new possibilities
Novel optical fibers based on photonic bandgap reflectors enable a new approach to beam delivery for medical CO2 lasers. A combination of desirable mechanical and optical properties allows flexible endoscopes to guide the laser beam into a patient’s body through a very small incision. For the first time, the advantageous high-precision tissue-cutting properties of the CO2 laser will become available for minimally invasive surgical procedures.
References
1. Yoel Fink et al (Nov. 27, 1998). SCIENCE, pp. 1679-1682.
2. Burak Temelkuran et al (Dec. 12, 2002). NATURE, pp. 650-653.
Meet the authors
Charalambos Anastassiou is measurements group leader; Gregor Dellemann, senior business development and product manager; Ori Weisberg, applications engineer; and Uri Kolodny, vice president of marketing and business development at OmniGuide Communications Inc. in Cambridge, Mass.