For scientists and astronomers building the optical components for telescopes, keeping pace with developing technology is presenting new challenges.
With a new generation of large telescopes comes new challenges for optics. Some mirrors, for instance, must be large but flexible to perform wavefront correction and make the images as clear as possible. The shape of future optics will be different from that of past telescopes, thanks to significant deviations from spherical curves. This improves performance but can make manufacturing and measurement challenging. These new telescopes also require mass production of precisely manufactured components.
The Extremely Large Telescope (ELT) being built at the European Southern Observatory (ESO) site in Chile is indicative of these trends. More than 90 percent of all of the contracts needed to make the telescope are in place (construction at the site began in May 2017).
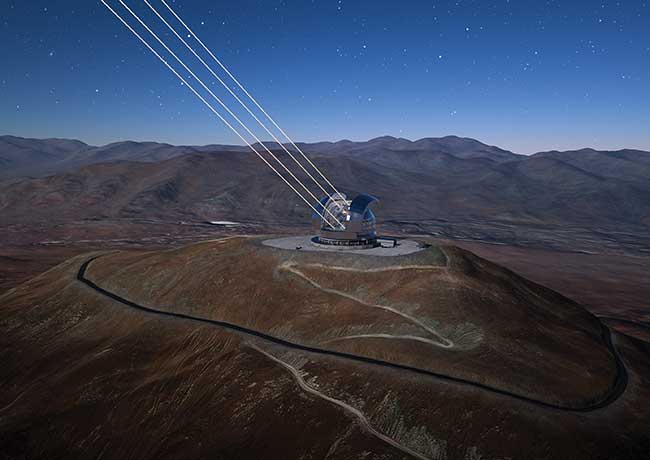
Depiction of adaptive optics at work in the European Extremely Large Telescope (ELT). Lasers create artificial guide stars in the atmosphere, which are used to flex a mirror’s surface to cancel out atmospheric turbulence, temperature fluctuations, and other effects. Courtesy of the European Southern Observatory/L. Calçada.
“This is a very significant milestone,” said Marc Cayrel, head of ELT optomechanics for ESO. He added that the telescope is on schedule for first light — the beginning of the instrument’s useful life — in 2024.
To hit that date, the project’s participants must manufacture many components in volume and to exacting specification. As is true for all modern large telescopes, the ELT’s primary mirror is made up of many smaller mirrors, with six sectors composed of 133 segments each in the 39-m-diameter primary mirror. There is also a backup sector, which is necessary to minimize downtime when the optics are recoated every year and a half or so. The backup segments will be swapped in, with the ones removed refurbished and made ready for the next time a swap is needed. Consequently, there are 931 segments, along with associated sensors and mechanics.
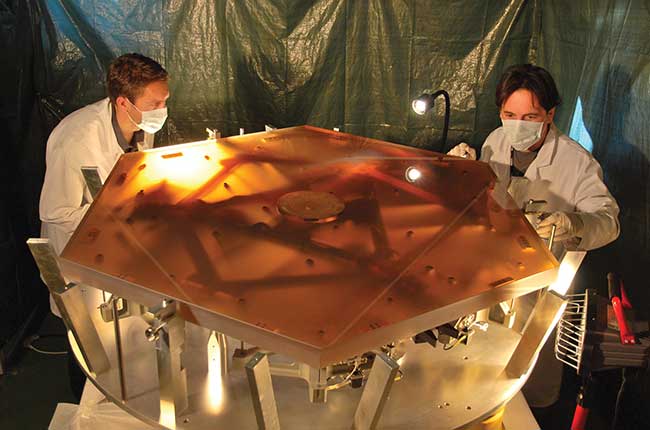
A prototype segment of the European ELT’s primary mirror, which was delivered in 2010 to demonstrate its manufacturing capability. Nearly 800 of these segments, each measuring about 1.5 m across, will be assembled to make up the 39-m-diameter primary mirror. Courtesy of Safran Reosc.
These optics and components must be produced at a peak rate of about one per day to meet the schedule, according to Cayrel. Each of the almost 1000 segments is a hexagonal, glass-ceramic mirror measuring about 1.5 m across. They are aspherical, and so have a surface profile that is not a part of a sphere. Aspherical optics shorten the focal length and significantly reduce the size — and cost — of the building that houses the telescope.
Primary mirrors
The segments of the primary mirror must be manufactured with a process that is reliable and repeatable. That minimizes risk while meeting specifications that are as tight as fractions of a wavelength. This manufacturing, in addition to the polishing, mounting, and integrating of the segments, is being handled by Safran Reosc, based in Saint-Pierre-du-Perray, France. According to Roland Geyl, vice president of business development, the goal is to achieve the required surface profile within 15 nm, at most.
“The process was fully demonstrated in 2010 with seven proto segments, the most difficult one being along the edge, delivered fully in spec,” he said. “The real challenge we are facing is the industrialization of this production up to one segment per day.”
Geyl noted that the company will exploit the industrial expertise of its parent company, Safran, which produces several, and much more complex, aircraft engines a day. He is confident the parts will be delivered on time, thanks to a self-funded internal study.
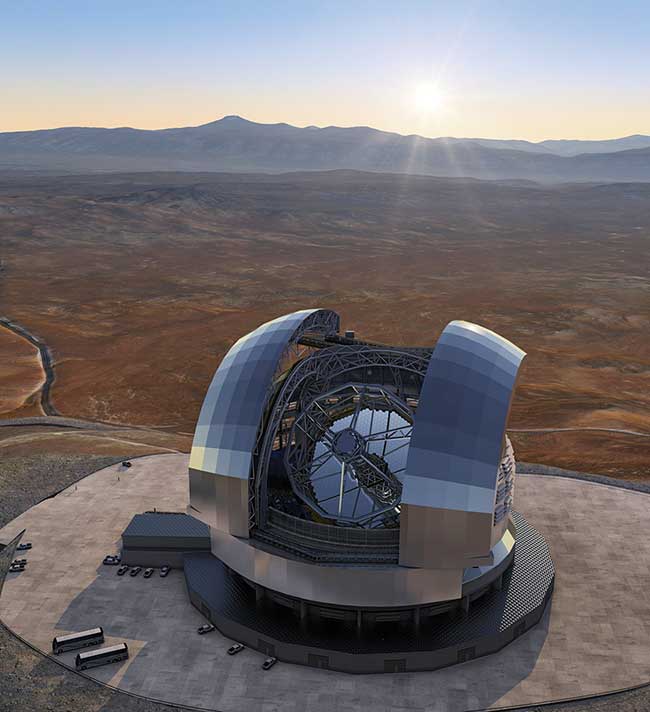
A rendering of the completed European ELT, which is being built on a site in Chile and will see first light in 2024. Courtesy of the European Southern Observatory.
According to ESO’s Cayrel, primary mirror segment manufacturing will take place in a 4000-sq-m facility. An industrial capability will be set up at the site in Chile to handle recoating of the segments when it becomes necessary. However, the primary mirror is only one of five in the ELT. The others, dubbed M2 through M5, are not segmented and must be crafted, polished, and mounted.
“These are pieces of engineering art. These are single pieces,” he said.
One challenge is that as the size of the individual pieces increases, the difficulty of fabrication also increases roughly to the third power, in part because temperature and other effects do not scale linearly. Thus, an optic that is twice the diameter of another is about eight times as difficult to make. On the other hand, important specifications that must be met do not change with size. For example, the diffraction limit is a function of the wavelength of light and so does not vary at all with increasing mirror size.
Achieving the diffraction limit requires eliminating the effects of atmospheric turbulence, which makes stars in the night sky twinkle. Correcting for this turbulence, and for the distorting effects of gravity and temperature on the shape of mirrors, makes the telescope produce as sharp an image as possible.
The needed correction is achieved with adaptive optics, a technology that flexes and bends a mirror’s surface to cancel out environmental and other effects. In the case of the ELT, this is handled by the
M4 mirror. The technology being installed will deform the mirror 1000 times per second, with 4500 actuators moving the mirror’s surface. It will be done in such a way as to hit the diffraction limit at the 2.2-µm wavelength, which is in the infrared region.
There are plans to push this wavelength down nearer to, and even into, the visible region, with this expected to take place in the late 2020s or early 2030s. This will improve the contrast and make it easier to detect and study planets circling other stars, Cayrel said. Moving down in wavelength will require changes to the adaptive optics — a greater density of actuators and each of these adjusting the mirror’s surface at a faster rate.
“In the future, it will be maybe more than 10,000 actuators, not running at
1 or 1.2 kHz but at 2 or 2.5 kHz,” he said. “[That is] so extreme in terms of density and in terms of speed.”
Further in the future is the possibility of using freeform optics. Aspheric optics deviate from the spherical by tens of millimeters over a span of meters, which requires special measurement and manufacturing methods. These have been developed and are now being used. Freeform optics, in contrast, radically departs from spherical shapes and thus requires research into and development of design techniques, measurement tools, and manufacturing capability. Freeform optics promises improved performance, lower costs, or both.
Trending technology
Two other telescopes being built or planned are examples of the trend toward — and challenges of — building big, higher-performance instruments. Instead of looking at the nighttime sky, however, these telescopes will study the nearest star: our sun.
One such telescope is the Daniel K. Inouye Solar Telescope, which will be operational in 2019. It is being built at the U.S. National Solar Observatory on Haleakala Mountain in Maui, Hawaii. The second is the European Solar Telescope, which will be deployed in the Canary Islands and is expected to see first light in 2027.
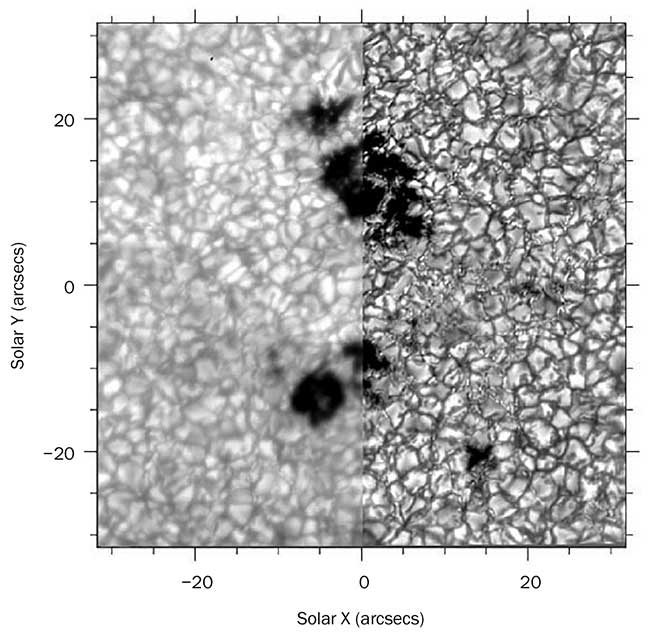
A solar image before (left) and after (right) speckle reconstruction, taken at the Richard B. Dunn Solar Telescope in New Mexico. The improvement in clarity can only be achieved by taking images rapidly, typically at 30 fps. The next generation of solar telescopes will be able to achieve 5× the spatial resolution. Courtesy of Queen’s University Belfast.
The telescopes will have a spatial resolution of as little as 20 km on the sun’s surface, thanks to large-format image sensors that will be at least 4K × 4K resolution. The detectors must be capable of handling high contrast, thereby making it possible to track the development of a bright flare as it evolves out of a relatively dark sunspot. The cameras also must run at a video or better frame rate, said Mihalis Mathioudakis, professor of astrophysics at Queen’s University Belfast in Northern Ireland, who is involved with the detectors for both projects.
The speed requirement derives from several factors, he said. One is that the smaller the structure seen on the sun, the faster it moves and evolves. So, the image capture time must be short to minimize motion artifacts. Aside from that, there is a need to collect as many as 50 images to produce one final high-resolution picture — because today’s adaptive optics technology cannot completely correct for atmospheric fluctuations in the UV range or at wavelengths below 400 nm.
The atmosphere changes too rapidly for current technology, so astronomers employ a technique known as speckle reconstruction. This approach uses many images to smooth out variations and thereby create an image as close as possible to the telescope’s resolution limit.
“The exposure times have to be very, very short,” Mathioudakis said. “They have to be of the order of a few milliseconds because you don’t want to have any fluctuations within your exposure time.”
This is being done using CMOS cameras from Belfast-based Andor Technology Ltd. These custom-developed image sensors have the necessary readout speed to handle data rates of several gigabytes per second. Fortunately, in solar observation there are plenty of photons involved, which makes fast imaging easier.
Nine of these cameras will go into the Daniel K. Inouye Solar Telescope for its initial setup. According to Mathioudakis, the European Solar Telescope will likely use backside illumination — a technology development expected over the next few years that will lead to higher efficiency in converting incoming photons into electrons. This will be an important improvement.
“As you increase the telescope’s aperture, where you can see smaller structures on the sun’s surface and the diffraction limit goes down,” Mathioudakis said, “you want to make use of every photon.”