Powerful, reliable, and compact, fiber lasers further offer the versatility to address a multitude of applications spanning a broad array of industries, and their potential continues to grow.
Bryce Samson, IPG Photonics Corporation
Early fiber lasers were inefficient and limited to low powers, until more effective methods emerged to deliver the pump light into the cladding. Valentin Gapontsev, founder and CEO of IPG Photonics, is credited with pioneering the use of distributed side-pumping into a large-area cladding by multiple single-emitter diode lasers, a method that overcame the limitations of previous pumping approaches. The side-pumping approach helped to unleash the true potential of fiber lasers, opened a new era of high-power fiber lasers and amplifiers, and revolutionized the further development of fiber technology. It also led to the mass adoption of fiber lasers in various industrial, scientific, and medical device applications.
Industrial fiber lasers have two stages, characterized by a power combiner and a brightness converter (Figure 1).
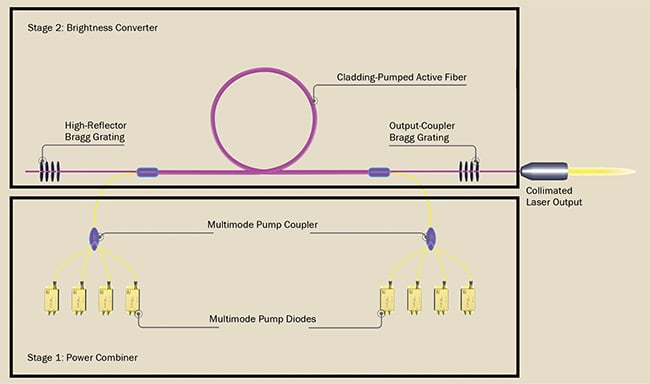
Figure 1. Industrial fiber lasers, such as this single-mode fiber laser configuration utilizing single-emitter diodes, have two stages characterized by a power combiner (Stage 1) and a brightness converter (Stage 2). Courtesy of IPG Photonics.
The power combiner in stage one comprises a collection of multiple laser diode pump packages designed to efficiently combine their multimode light into a passive transmission fiber. The use of a redundant number of single-emitter diode packages ensures high reliability of the laser. The laser’s optical cavity, defined by two fiber Bragg grating mirrors, is located in the central single-mode core, a cladded high-purity optical fiber doped with a variety of rare-earth elements.
The cavity converts the low-quality diode light into single-mode laser light. One of the fiber Bragg gratings acts as a total reflector, while the other serves as a partial reflector or output coupler. The multimode cladding is not doped. It serves only to propagate the diode pump light. The solid-state architecture of fiber lasers makes them insusceptible to environmental factors such as dust, moisture, and free-space air perturbations.
The overall pumping method is more than 50% electrically efficient and produces single-mode output of around 2 to 3 kW of continuous-wave power from a single module. Outputs of individual modules can be used directly or combined to deliver high brightness outputs exceeding 100 kW, which makes fiber lasers capable of addressing a variety of industrial applications (Figure 2).
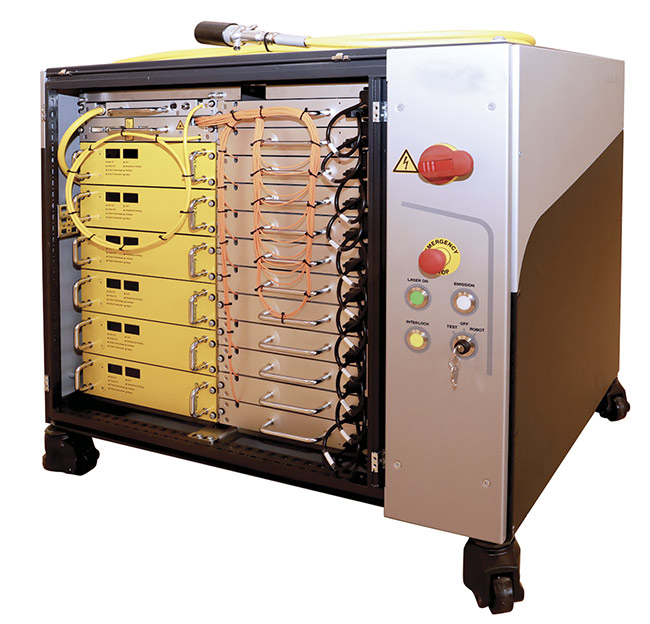
Figure 2. Kilowatt-class fiber laser systems combine multiple single-mode modules in parallel, to output a single beam through a larger-core-diameter step-index fiber. Courtesy of IPG Photonics.
Mode of operation
Fiber lasers can be classified by several operation modes, including continuous wave (CW), quasi-CW (QCW), nanosecond pulsed, and ultrafast pico- or femtosecond pulsed.
CW lasers provide steady output within a maximum specified output power. They can be modulated up 50 kHz depending on output power, but modulation does not increase their peak power. CW lasers find use in a multitude of applications — most notably for cutting and welding metals but also for brazing, 3D printing, cladding, and heat treating.
Long pulses from 10 QCW lasers can increase both pulse energy and peak output power by a factor of 10, with long pulse durations extending from about 10 to 100,000 µs. A QCW laser operating at 300-W average power, for example, provides 3 kW of peak power with a pulse energy of 30 J.
QCW lasers are primarily used in welding and drilling applications, as well as in specialty cutting operations, such as those for cutting highly reflective metals or other materials. Standard QCW models range from 1 to 20 kW in peak power, and operate at a substantially lower cost than competing laser technologies with comparable output.
Nanosecond-pulsed Q-switched fiber lasers provide a range of average output powers, from 10 W to 2 kW. Pulse durations can either be fixed or adjustable (preprogrammed, selectable by the user) within the 1- to 1000-ns range. Typical pulse energies for lasers in the 10- to 300-W range, with the near single-mode beam quality used in microprocessing, can be up to approximately 1 mJ. These lasers can be modulated to operate in the kilohertz to megahertz range, depending on the model. Pulsed lasers with the higher average power used for high-speed surface processing achieve pulse energies up to 100 mJ and deliver a larger processing spot.
Ultrafast picosecond and femtosecond fiber lasers offer pulse durations from 200 fs to several picoseconds and average powers ranging from 10 to 200 W. They are used in a variety of microprocessing applications, including metals and nonmetals.
Wavelength range
A fiber laser’s active lasing core can be doped with one or more active atoms to generate standard outputs in one of several spectral ranges (Figure 3). Doping with ytterbium (Yb) atoms, for example, generates outputs between 1030 and
1080 nm; erbium (Er) produces wavelengths between 1500 and 1570 nm; and thulium (Tm) produces 1900- to 2050-nm light. These fundamental lines can be frequency doubled or tripled, giving
rise to lasers emitting green (515- to
550-nm) and UV (~355-nm) beams. Raman shifting of the fundamental
ytterbium and erbium wavelengths extends their accessible range to between 1.15 and 1.8 µm. Further frequency doubling of these wavelengths allows fiber lasers to operate in the visible range from 515 to 635 nm. Additionally, hybrid solid-state lasers pumped by CW thulium or erbium fiber lasers provide output in the mid-IR range between 1.9 and >5 µm.
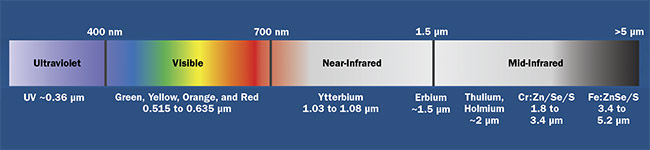
Figure 3. A fiber laser’s active lasing core can be doped with one or more active atoms to generate standard outputs in one of several spectral ranges. Courtesy of IPG Photonics.
Beam spatial modes
Fiber lasers can be configured with a variety of spatial beam modes to fit virtually any application. These modes are a property of the single-mode fiber used, rather than a thermal operating point. Consequently, fiber lasers, unlike other solid-state lasers, produce the same beam profile, with no change in divergence over their entire operating power range, typically from 10% to 100% of specified power.
Single-mode CW lasers with near transform-limited beam quality are available for industrial applications requiring average powers up to 10 kW and minimum spot sizes in the 25- to 30-µm range. High-brightness multimode lasers have output fiber core diameters in the 50- to 600-µm range with beam parameter product values starting at 2 mm×mrad. The output intensity distribution can be bell-shaped or flat-topped from an up to 1-mm core diameter (Figure 4).
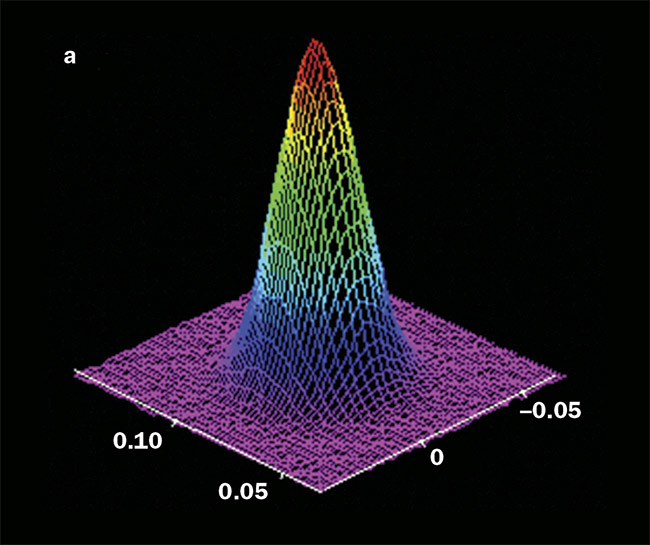
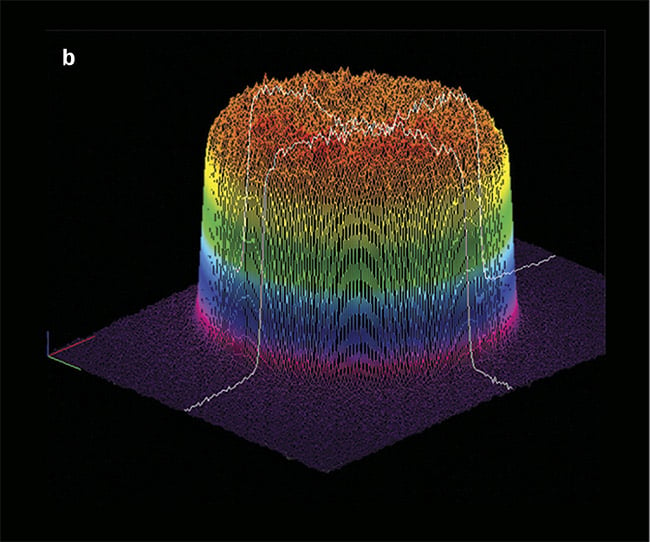
Figure 4. The output intensity distribution of fiber lasers can exhibit a bell-shaped single-mode profile (a) or a flat-topped multimode profile (b). Courtesy of IPG Photonics.
Particular applications may require further spatiotemporal intensity distribution through the use of a dual-spot module, trifocal beam delivery, beam wobbling, or adjustable mode beam (AMB) solutions. AMB lasers feature a coaxial ring around a fiber laser’s inner core. Power levels in the core and the ring can be independently adjusted and changed on the fly. AMB lasers improve performance in drilling, cutting, and welding. They are particularly effective for the welding of aluminum, in which the additional ring energy stabilizes the welding keyhole, reducing or completely eliminating spatter, seam cracking, and porosity (Figure 5).
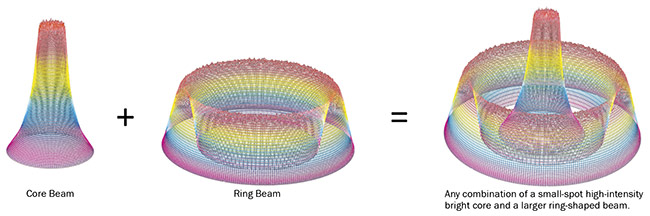
Figure 5. Adjustable-mode beam profile solutions enable fiber lasers to feature a coaxial ring around a fiber laser’s inner core, both of which can be independently adjusted and changed on the fly to improve performance in materials processing applications. Courtesy of IPG Photonics.
Applications and benefits
Since the early 1990s, fiber lasers have established themselves in the telecom and medical procedure markets, as well as in a variety of advanced and scientific applications. The wide wavelength range, narrow linewidths, polarized or unpolarized emissions, short pulse durations, single-mode operation, insensitivity to environmental conditions, and compact size of fiber lasers make them a favorable solution for the scientific and government communities, where the lasers often help to solve challenges that other laser technologies cannot.
During the 2000s, industrial fiber lasers were increasingly used for processing materials in applications across automotive, aerospace, heavy industry and transport, consumer devices, electronics, medical devices, oil and gas, nuclear power, photovoltaics, semiconductor manufacturing, and other industries.
While materials processing of metals has accounted for the bulk of early adoption, other quickly emerging applications include cladding and 3D printing, heat treating, surface cleaning and modification, and a variety of microprocessing techniques encompassing polymer,
ceramic, and other nonmetal materials.
Across all of these industries and
applications, industrial fiber lasers have emerged as a benchmark for performance. Their comparably higher output powers and uniformly excellent beam quality help to ensure fast processing speeds, while their resistance to vibration and contamination, rugged compact packaging, efficient energy consumption, and reliability contribute to a fast return on investment.
Meet the author
Bryce Samson is director of North American sales for IPG Photonics, where he has worked for nearly five years. He has worked in the field of fiber lasers since 1996 and is the
co-author of the book Fiber Lasers: Basics, Technology and Applications (CRC Press, 2016).
Acknowledgments
This article draws on numerous fiber laser articles written by Bill Shiner, who was vice president of industrial sales at IPG for almost two decades and who passed away May 7, 2020. The article has been updated with recent advancements in the field by several of Shiner’s colleagues at IPG, while maintaining the core of his contribution to the topic.