Mar. 19, 2025
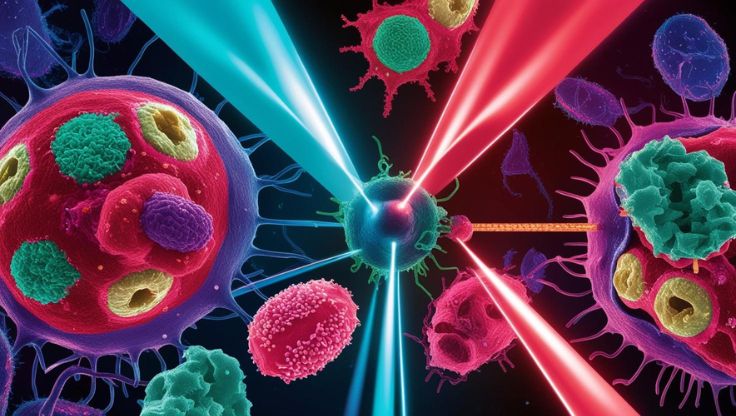
Raman Spectroscopy
Raman spectroscopy has blossomed into a pivotal analytical technique, transforming fields from medical diagnostics to environmental surveillance. The emergence of compact transmission-based Raman spectrometers heralds a new era of analytical excellence, characterized by unmatched portability, efficiency, and stability. These devices facilitate rapid, non-invasive molecular analysis, rendering them indispensable in applications such as early-stage disease detection, environmental pollutant monitoring, and stringent quality control in the food and pharmaceutical sectors. This article delves into the technological advancements and pragmatic applications of compact transmission-based Raman spectrometers, showcasing their profound impact and potential to spearhead future innovations. By integrating advanced techniques and combining Raman with Laser-Induced Breakdown Spectroscopy (LIBS) or Fluorescence Spectroscopy, these spectrometers are poised to redefine analytical paradigms. These synergistic approaches enhance the detection capabilities, providing comprehensive elemental and molecular information and paving the way for groundbreaking discoveries in planetary research and beyond.
Key Technologies: Raman spectroscopy, laser-induced breakdown spectroscopy, fluorescence spectroscopy
Brillouin Microscopy
Brillouin Microscopy is a label-free, non-contact technique that relies on the inelastic scattering of light to probe the viscoelastic properties of materials, including various biomaterials such as cells and tissues. Understanding the mechanical properties of cells and tissues is vital for understanding how cells differentiate, how stem cells choose a lineage during proliferation, and diseases progress. When probing soft biological materials, a non-contact approach is often favourable as many contact approaches are better used with more rigid inorganic materials (nanoindentation for example) and they will often destroy the sample. The article will look at why Brillouin microscopy is being used, the underlying principles of how Brillouin microscopy works, why there’s an importance for understanding the mechanical properties of biomaterials, and what some of the key applications are, including a deeper dive into how Brillouin microscopy is playing an important role in neuromuscular junction research (where motor neurons connect with muscle fibres).
Key Technologies: Brillouin microscopy, atomic force microscopy, optical elastography, electron microscopy
Optical Filters
Modern interference-coated filters are currently pushing boundaries in a number of biomedical applications using regions of the electromagnetic spectrum not historically used in imaging. In particular, the region commonly known as “SWIR” (short wavelength infrared, generally ranging from ~900-2500nm) has become the focus in these approaches. Most of these applications require very efficient, relatively narrow transmission bands with high degrees of out-of-band blocking over a wide range of wavelengths (including most of the “visible” range) to maintain signal integrity. Use of the SWIR range in cutting-edge instrumentation allows for analyses running the gamut from point-of-care to orbital platforms, and includes: assisting in surgical suites to improve marginal contrast in glioblastoma tumor removal, performing hyperspectral imaging of entire agricultural crops to survey distribution and health of the growing plants, and remote sensing and quality control of large-volume pharmaceutical and food products as they are prepared for market. This article will discuss some of these applications and current efforts to optimize filter production to support these burgeoning fields.
Key Technologies: Optical filters, SWIR, InGaAs arrays, lasers, LEDs
Light Therapy
Recent advancements in laser technology have opened new possibilities in the production of radioisotopes for cancer treatments. Traditional methods of isotope production rely on nuclear research reactors or cyclotrons, each with its limitations: cyclotrons can only produce a subset of needed isotopes, while ageing reactors face increasing infrastructure challenges.
The laser-based method, described in this research, introduces an innovative and more flexible approach by using high-intensity femtosecond laser pulses to drive nuclear reactions. This process can generate different short-lived isotopes through targeted and reconfigurable schemes, opening a promising new way of producing isotopes for imaging or therapy thanks to the never-ending improvements of ultrafast laser technology.
The potential impact on cancer treatment is profound: more targeted therapy, fewer patient side effects, and a more sustainable approach to radioisotope production. This innovation could pave the way for more accessible and effective treatments across the globe.
Key Technologies: Lasers, laser therapy
Download Media Kit